CR2.4
Fell January 15, 1824
44° 46′ N., 11° 17′ E. At 8:30 P.M. local time in Renazzo, Italy, a bright light was seen and three detonations heard by residents as several stones fell. Three individual stones were recovered having a combined weight of 10 kg, the largest weighing ~5 kg. Renazzo is a brecciated meteoriteWork in progress. A solid natural object reaching a planet’s surface from interplanetary space. Solid portion of a meteoroid that survives its fall to Earth, or some other body. Meteorites are classified as stony meteorites, iron meteorites, and stony-iron meteorites. These groups are further divided according to their mineralogy and Click on Term to Read More with a shock stageA petrographic assessment, using features observed in minerals grains, of the degree to which a meteorite has undergone shock metamorphism. The highest stage observed in 25% of the indicator grains is used to determine the stage. Also called "shock level". Click on Term to Read More ranging from S1 to S3. This meteorite is a preserved fallMeteorite seen to fall. Such meteorites are usually collected soon after falling and are not affected by terrestrial weathering (Weathering = 0). Beginning in 2014 (date needs confirmation), the NomComm adopted the use of the terms "probable fall" and "confirmed fall" to provide better insight into the meteorite's history. If Click on Term to Read More with a unique volatileSubstances which have a tendency to enter the gas phase relatively easily (by evaporation, addition of heat, etc.). elementSubstance composed of atoms, each of which has the same atomic number (Z) and chemical properties. The chemical properties of an element are determined by the arrangement of the electrons in the various shells (specified by their quantum number) that surround the nucleus. In a neutral atom, the number of Click on Term to Read More and N-isotopic composition that distinguishes it from the other carbonaceous chondriteCarbonaceous chondrites represent the most primitive rock samples of our solar system. This rare (less than 5% of all meteorite falls) class of meteorites are a time capsule from the earliest days in the formation of our solar system. They are divided into the following compositional groups that, other than Click on Term to Read More groups. It serves as the type specimen of the Renazzo-type chondritesChondrites are the most common meteorites accounting for ~84% of falls. Chondrites are comprised mostly of Fe- and Mg-bearing silicate minerals (found in both chondrules and fine grained matrix), reduced Fe/Ni metal (found in various states like large blebs, small grains and/or even chondrule rims), and various refractory inclusions (such Click on Term to Read More, which are considered to be among the least thermally altered of the known meteorite groups.
Diagram adapted from Schrader et al. (and references therein), MAPS, vol. 50, #1, p. 37 (2015)
‘The formation and alteration of the Renazzo-like carbonaceous chondrites III: Toward understanding the genesis of ferromagnesian chondrules’
(http://dx.doi.org/10.1111/maps.12402) The matrixFine grained primary and silicate-rich material in chondrites that surrounds chondrules, refractory inclusions (like CAIs), breccia clasts and other constituents. Click on Term to Read More component in Renazzo, which includes igneous fragments, isolated mineralInorganic substance that is (1) naturally occurring (but does not have a biologic or man-made origin) and formed by physical (not biological) forces with a (2) defined chemical composition of limited variation, has a (3) distinctive set of of physical properties including being a solid, and has a (4) homogeneous Click on Term to Read More grains, CAIsSub-millimeter to centimeter-sized amorphous objects found typically in carbonaceous chondrites and ranging in color from white to greyish white and even light pink. CAIs have occasionally been found in ordinary chondrites, such as the L3.00 chondrite, NWA 8276 (Sara Russell, 2016). CAIs are also known as refractory inclusions since they Click on Term to Read More, AOAs, dark inclusions, and metalElement that readily forms cations and has metallic bonds; sometimes said to be similar to a cation in a cloud of electrons. The metals are one of the three groups of elements as distinguished by their ionization and bonding properties, along with the metalloids and nonmetals. A diagonal line drawn Click on Term to Read More grains, accounts for ~35–40 vol% of the bulk composition (Bayron et al., 2014). The abundance of chondrulesRoughly spherical aggregate of coarse crystals formed from the rapid cooling and solidification of a melt at ~1400 ° C. Large numbers of chondrules are found in all chondrites except for the CI group of carbonaceous chondrites. Chondrules are typically 0.5-2 mm in diameter and are usually composed of olivine Click on Term to Read More and chondruleRoughly spherical aggregate of coarse crystals formed from the rapid cooling and solidification of a melt at ~1400 ° C. Large numbers of chondrules are found in all chondrites except for the CI group of carbonaceous chondrites. Chondrules are typically 0.5-2 mm in diameter and are usually composed of olivine Click on Term to Read More fragments in Renazzo is ~50–60 vol%. Multilayered, FeO-poor (type-I) chondrules (mostly porphyritic attesting to partially melting) constitute the vast majority of the chondrules, with the remainder consisting of sulfide-rich, FeO-rich (type-II) chondrules and chondrule fragments. Rare relict type-I grains (and/or compositionally similar precursor material) have been identified within some type-II chondrules (Schrader et al., 2015). Al-rich chondrules have also been identified in minor abundances. The chondrules, metal, and matrix formed under variable conditions from a common nebulaAn immense interstellar, diffuse cloud of gas and dust from which a central star and surrounding planets and planetesimals condense and accrete. The properties of nebulae vary enormously and depend on their composition as well as the environment in which they are situated. Emission nebula are powered by young, massive Click on Term to Read More reservoir. This is attested by the complimentary chemical composition of these components, as well as by the enrichment and depletion in metal and silicateThe most abundant group of minerals in Earth's crust, the structure of silicates are dominated by the silica tetrahedron, SiO44-, with metal ions occurring between tetrahedra). The mesodesmic bonds of the silicon tetrahedron allow extensive polymerization and silicates are classified according to the amount of linking that occurs between the (respectively) of the same presolar s-process carrier phase (observed as a correlation in nucleosynthetic Mo and W isotopeOne of two or more atoms with the same atomic number (Z), but different mass (A). For example, hydrogen has three isotopes: 1H, 2H (deuterium), and 3H (tritium). Different isotopes of a given element have different numbers of neutrons in the nucleus. Click on Term to Read More anomalies) (Budde et al., 2018). All of these components ultimately agglomerated in the outer Solar SystemThe Sun and set of objects orbiting around it including planets and their moons and rings, asteroids, comets, and meteoroids. (beyond Jupiter) at approximately the same heliocentricCentered around a sun. Our own Solar System is centered around the Sun so that all planets such as Earth orbit around the Sun. Note that 25% of Americans incorrectly believe the Sun revolves around the Earth. Click on Term to Read More distance as the CI chondrites. Based on the initial 26Al/27Al ratios calculated for chondrules from unequilibrated ordinary chondrites and CO3.0 chondrites, they are considered to have formed 1–2 m.y. after CV CAIs (age = ~4.567 b.y.). By contrast, the lower initial 26Al/27Al ratios inferred for chondrules in CR chondrites are consistent with a relatively late formation of ~2.5 m.y. after CAIs (Scott et al., 2007; Nagashima et al., 2008). A similar age of 2.5 (±0.9) m.y. after CAIs was determined by Amelin et al. (2002) utilizing the Pb–Pb dating method, which was translated by Connelly et al. (2012) to 3.66 (±0.63) m.y. after CAIs by employing a corrected 238U/235U ratio. The Pb–Pb chronometer was also applied to the CR chondriteClass named for the Renazzo meteorite that fell in Italy in 1824, are similar to CMs in that they contain hydrous silicates, traces of water, and magnetite. The main difference is that CRs contain Ni-Fe metal and Fe sulfide that occurs in the black matrix and in the large chondrules Click on Term to Read More NWA 6043, and it revealed a wide range of ages—from the oldest known chondrule at 4.5673 (±0.0010) b.y., to chondrules as much as 3.64 m.y. younger (Bollard et al., 2014). They reasoned that the latest formation of chondrules 4.56366 (±0.00091) b.y. ago establishes a benchmark for the time of dissipation of the solar accretion diskDisk-shaped cloud of gas and solids in orbital motion around a central protostar or some other massive central body. Click on Term to Read More. In a comprehensive study of CR chondrules by Schrader et al. (2016) that included Al–Mg systematics, they recognized three distinct populations of chondrules dated at 2.2 (+0.1/–0.2), 2.9 (+0.2/–0.2), and 4.4 (+0.7/–0.4) m.y. after CV CAIs; the latter oldest age represents the largest population in the study. They concluded that accretionAccumulation of smaller objects into progressively larger bodies in the solar nebula leading to the eventual formation of asteroids, planetesimals and planets. The earliest accretion of the smallest particles was due to Van der Waals and electromagnetic forces. Further accretion continued by relatively low-velocity collisions of smaller bodies in the Click on Term to Read More of the CR parent body continued for at least ~4.0 m.y. after formation of the earliest solids in the solar nebulaThe primitive gas and dust cloud around the Sun from which planetary materials formed. as represented by CAIs in CV chondritesMeteorite class named after the Vigarano meteorite that fell in Italy in 1910. They have abundant large, well-defined rimless (?) chondrules of magnesium-rich olivine (~0.7 mm diameter; 40-65 vol. %), often surrounded by iron sulfide. They also contain 7-20 vol. % CAIs. The often dark-gray matrix is dominated by Fe-rich Click on Term to Read More. Moreover, their study indicates that 26Al was uniformly distributed in the CR chondriteChondrites are the most common meteorites accounting for ~84% of falls. Chondrites are comprised mostly of Fe- and Mg-bearing silicate minerals (found in both chondrules and fine grained matrix), reduced Fe/Ni metal (found in various states like large blebs, small grains and/or even chondrule rims), and various refractory inclusions (such Click on Term to Read More formation region. From a weighted mean of 21 CR chondrules, Schrader et al. (2017) obtained a preferred Al–Mg age of 3.75 (±0.24) m.y. after CAIs. A high-precision Hf–W isotopic analysis of Renazzo and three other CR chondrites was conducted by Budde et al. (2018) in order to constrain the timing of chondrule formation, accretion, and metal–silicate segregation for the CR parent body. They determined a Hf–W age of 3.6 (±0.6) m.y., which is in agreement with that determined by the Pb–Pb and Al–Mg chronometers. In addition, they ascertained a weighted mean age based on all three chronometers of 3.73 (±0.21) m.y. after CAIs. Utilizing Mn–Cr systematics, Trinquier et al. (2008) calculated an equilibriumTerm used to describe physical or chemical stasis. Physical equilibrium may be divided into two types: static and dynamic. Static equilibrium occurs when the components of forces and torques acting in one direction are balanced by components of forces and torques acting in the opposite direction. A system in static Click on Term to Read More age for CR2 chondrites of between 1.1 and 6.2 m.y. after CAIs. Because the CR chondrules were accreted into the developing parent body after most of the radiogenic 26Al had decayed, the degree of thermal metamorphism was limited. Among other petrographic features, the high abundance of Cr2O3 that remains in the metal phase attests to this low degree of thermal metamorphism (Wasson and Rubin, 2010; Schrader et al., 2015 [see diagram above]). Jilly-Rehak et al. (2016) conducted new 53Mn–53Cr analyses of secondary carbonates—calcite in the matrices of Renazzo and GRO 95577 and of dolomite in a Renazzo dark inclusionFragment of foreign (xeno-) material enclosed within the primary matrix of a rock or meteorite. Click on Term to Read More. They determined aqueous alteration ages anchored to the D’Orbigny angriteType of evolved achondrite meteorite that represent some of the earliest stages of asteroidal differentiation and magmatism in our solar system. Angrites are named for the Angra dos Reis meteorite, which fell in Rio de Janeiro, Brazil, in early 1869. They are basaltic (mafic) rocks, often containing porous areas, and Click on Term to Read More of 4.5634 (+0.0028/–0.0074) b.y. for Renazzo calcite, and 4.5554 (+0.0014/–0.0021) b.y. for GRO 95577 calcite; these ages correspond to 4.6 (+7.4/–2.8) m.y. and 12.6 (+2.1/–1.4) m.y. after CV CAIs (4.56794 [±0.00031] b.y.; Bouvier et al., 2011), respectively. The prolonged period of aqueous alteration revealed by this study indicates that impact shock was necessarily a significant heating mechanism on the CR parent body following the cessation of radiogenic heating from 26Al decay. A basic scenario for the early petrogenesis of Renazzo has been described. Initially, Ti-bearing perovskiteTerm applied to A2+B4+O3 high-pressure minerals with a perovskite structure (general formula ABX3) where "A" is a metal that forms large cations such as Mg, Fe or Ca, "B" is another metal that forms smaller cations such as Si (called silicate perovskite), Ti and to a lesser degree Al, and Click on Term to Read More condensates facilitated the condensation of forsteritePure* magnesium end-member (Mg2SiO4) of the olivine solid solution series and an important mineral in meteorites. When magnesium (Mg) is completely substituted by iron, it yields the the pure Fe-olivine end member, fayalite (Fe2SiO4). The various Fe and Mg substitutions between these two end-members are described based on their forsteritic (Fo) Click on Term to Read More. Lower temperatures and more highly reducingOxidation and reduction together are called redox (reduction and oxidation) and generally characterized by the transfer of electrons between chemical species, like molecules, atoms or ions, where one species undergoes oxidation, a loss of electrons, while another species undergoes reduction, a gain of electrons. This transfer of electrons between reactants Click on Term to Read More conditions prevailed as small low-Ni, FeNi-metal grains and fine-grained pyroxeneA class of silicate (SiO3) minerals that form a solid solution between iron and magnesium and can contain up to 50% calcium. Pyroxenes are important rock forming minerals and critical to understanding igneous processes. For more detailed information, please read the Pyroxene Group article found in the Meteoritics & Classification category. Click on Term to Read More dust combined to form aggregates. Thereafter, these precursor coarse-grained aggregates were melted by a solar heat pulse and then cooled from peak temperatures at rates of 0.9–96.7 K/hour. These conditions are more consistent with nebula shock wave-induced heating rather than through lightning or the x-wind (Chaumard et al., 2015). These melted aggregates ultimately coalesced to form the Ti-enriched, FeO-poor, porphyritic type-I chondrules composing Renazzo. Nebular-condensed C was dissolved in the metal and later exsolved in the newly formed chondrules (Kong et al., 1999). Contemporaneously, mineral assemblages comprising FeNi-metal, sulfides (pentlanditeFe-Ni sulfide, (Fe,Ni)9S8, that is often associated with troilite, and found in the matrix and chondrules of CO, CV, CK and CR chondrites. The color is yellow-bronze with light bronze-brown streak and metallic luster. It typically forms during cooling of magmatic sulfide melts during the evolution of parent silicate melt. The Click on Term to Read More and pyrrhotiteIron sulfide group of minerals whose composition ranges widely between its end members pyrrhotite (Fe7S8) whose crystal structure is monoclinic, and troilite (FeS) whose crystal structure is hexagonal. Its general formula is Fe1−xS (where x = 0 to 0.17). The troilite phase is found mainly in meteorites and in the Click on Term to Read More), and phosphate were formed in the aftermath of high-temperature gas–solid sulfidation processes in the solar nebula (Schrader et al., 2008, 2015). Igneous rims composed of silica-rich pyroxene are present on most CR type-I chondrules (although not evident in Renazzo or Al Rais due to their higher degree of aqueous alteration). These rims are presumed to have formed by direct condensation of silicaSilicon dioxide, SiO2. onto chondrule surfaces from a cooling, fractionated nebular gas (Krot et al., 2003, 2004). FeNi-metal occurs along the rims of a significant portion (~40%) of type-I chondrules in CR chondrites, and also occurs both in chondrule interiors and as isolated grains in interchondrule matrix material. The amoeboid-shaped metal grains on chondrule rims were once considered to have formed by migration of FeNi-metal grains from chondrule interiors through centrifugal forces associated with rapid spinning of these chondrules (Grossman and Wasson, 1985; Kong and Palme, 1999); however, the distribution of the metal bears more resemblence to a discontinuous shell than a ring. Noting the minor effects centrifugal forces may have, it was argued by Wasson and Rubin (2009) that under high temperature conditions, with the molten metal having lower surface tension with respect to the vacuumSpace entirely devoid of matter (called also, by way of distinction, absolute vacuum). In a more general sense, a space, as the interior of a closed vessel, which has been evacuated to a high degree by a pump or other artificial means. of space than to the molten silicates, metal was induced to form along the outer surface. Upon cooling, the interface tension became more influential than the surface tension, causing the metal to take the form of small globules. The formation of metal in CR chondrites was examined in-depth by Jacquet et al. (2013, 2014), who assessed the likelihood of the four competing theories: 1) direct condensation from the nebula, 2) silicate reductionOxidation and reduction together are called redox (reduction and oxidation) and generally characterized by the transfer of electrons between chemical species, like molecules, atoms or ions, where one species undergoes oxidation, a loss of electrons, while another species undergoes reduction, a gain of electrons. This transfer of electrons between reactants Click on Term to Read More processes, 3) evaporationProcess in which atoms or molecules in a liquid state (or solid state if the substance sublimes) gain sufficient energy to enter the gaseous state. Click on Term to Read More/recondensation, and 4) desulfurization of FeS. A brief synopsis of their scenario is presented below (∗). A fifth mechanism has been proposed by Chaumard et al. (2014) to describe the formation of an isolated, igneously-zoned, mm-sized matrix metal grain in Renazzo—fractional crystallizationA crystallization process in which minerals crystallizing from a magma are isolated from contact with the liquid. It is a key process in the formation of igneous rocks during the process of magmatic differentiation. Also known as crystal fractionation. Click on Term to Read More of a molten droplet with subsequent recondensation of volatile siderophile elements on the exterior margin which diffused inwards during cooling. Utilizing 3-D microtomographic imaging of Renazzo chondrules, Ebel et al. (2009) and Ebel and Downen (2011) discovered that some chondrules exhibit multiple discrete, concentric, metallic layers alternating with silicate layers, suggesting sequential accretion of independent metal and silicate components. These represent sequential generations of chondrule formation attributable to multiple local heating events within the same unique nebular source region. These accretionary periods were followed by intervals of rapid annealing, which occurred in a cooling disk environment under reducing conditions. During this period, impacts produced a petrofabric in the form of chondrule flattening in this matrix-rich (31 vol%) meteorite, resulting in a chondrule axial ratio of ~1.3 (Kallemeyn et al., 1994). The finer-grained matrix material was also formed in this same Ti-depleted nebular region, but only after substantial cooling had occurred. During this time, abundant water ices that had accreted along with the matrix material promoted the formation of phyllosilicatesClass of hydroxyl-bearing silicate minerals with a sheet-like structure. They result from aqueous alteration are dominantly serpentine and smectite in meteorites; found in the matrixes of carbonaceous chondrites. Phyllosilicates consist of repeating sequences of sheets of linked tetrahedra (T) and sheets of linked octahedra (O). The T sheet consists of Click on Term to Read More. All of these various components constituting the CR parent body agglomerated in a geological instant, at a time ~2.5 m.y. after CAISub-millimeter to centimeter-sized amorphous objects found typically in carbonaceous chondrites and ranging in color from white to greyish white and even light pink. CAIs have occasionally been found in ordinary chondrites, such as the L3.00 chondrite, NWA 8276 (Sara Russell, 2016). CAIs are also known as refractory inclusions since they Click on Term to Read More formation. It was demonstrated that if the bulk chemical compositions of both the highly variable population of Renazzo chondrules and the matrix materials are calculated together, they preserve the solar elemental abundance ratios. This complementarity indicates that the accretion process of CR chondrites probably occurred as a closed systemDefinable part of the universe that can be open, closed, or isolated. An open system exchanges both matter and energy with its surroundings. A closed system can only exchange energy with its surroundings; it has walls through which heat can pass. An isolated system cannot exchange energy or matter with within a unique chondritic region of the protoplanetary diskFlattened and rotating disk of dense gas and dust/solids orbiting a young star from which planets can eventually form. Click on Term to Read More (Ebel et al., 2009). The much rarer FeO-rich (type-II) chondrules present in CR chondrites lack accretionary rims, exhibit mostly broken surfaces, and some contain relict grains with Fa values and O-isotopic ratios indicative of recycling from an earlier generation of type-I chondrules (Connolly et al., 2003, 2008). They exhibit a wide range of bulk FeO and O-isotopic compositions and have a heavier O-isotopic signature than that of type-I chondrules. It is considered that the gas may have evolved from reducing to highly oxidizingOxidation and reduction together are called redox (reduction and oxidation) and generally characterized by the transfer of electrons between chemical species, like molecules, atoms or ions, where one species undergoes oxidation, a loss of electrons, while another species undergoes reduction, a gain of electrons. This transfer of electrons between reactants Click on Term to Read More during the interval between type-I and type-II chondrule formation. In addition, the O-isotopic signature evolved from 16O-rich to 16O-poor; this enrichment of heavy oxygenElement that makes up 20.95 vol. % of the Earth's atmosphere at ground level, 89 wt. % of seawater and 46.6 wt. % (94 vol. %) of Earth's crust. It appears to be the third most abundant element in the universe (after H and He), but has an abundance only Click on Term to Read More isotopes was due to the evaporation of water ice, which also created a more highly oxidizing environment (Connolly and Huss, 2010). The O-isotopic composition of type-II chondrules overlaps that of ordinary chondrites, suggesting a complex formation history in an oxidizing and sulfidizing environment. After an episode of fragmentation, which was possibly associated with the accretion of the CR parent body, the type-II chondrules were subjected to impact heating and aqueous alteration from previously accreted water ices. Consistent with its content of magnetiteFe oxide, Fe2+Fe3+2O4, containing oxidized iron (Fe3+) found in the matrix of carbonaceous chondrites and as diagnostic component in CK chondrites. In CK chondrites, magnetite is typically chromian, containing several wt. % Cr2O3. Click on Term to Read More, Renazzo reflects a greater degree of aqueous alteration than many CR group members. Aside from its brecciated structure, the extent of aqueous alteration is responsible for a porosityThe volume percentage of a rock that consists of void space. Vesicular porosity is a type of porosity resulting from the presence of vesicles, or gas bubbles, in igneous rock such as the pumice presented here. Vesicular porosity is very rare in meteorites and is often associated with slag, one Click on Term to Read More that ranges from 3.7% to 18.2% (Macke et al., 2011). Results of trace-element studies have identified primitive glass inclusions within olivines that sample the liquid–vapor barrier that lead to olivine formation. These inclusion glasses formed contemporaneously with the host olivine in a dust- and oxygen-enriched region of the condensing nebula. These primary glass inclusions are found to be either Al-rich and derived from unfractionated nebular condensates, Al-poor and derived through fractionationConcentration or separation of one mineral, element, or isotope from an initially homogeneous system. Fractionation can occur as a mass-dependent or mass-independent process. Click on Term to Read More and removal of refractory components from the nebula vapor, or Na-rich and derived from Al-rich parental glass after a metasomatic (solid–vapor) exchange of Ca for Na in the nebula (Varela et al., 2001). Presolar grainsMineral grains that formed before our solar system. These tiny crystalline grains are typically found in the fine-grained matrix of chondritic (primitive) meteorites. Most grains probably formed in supernovae or the stellar outflows of red giant (AGB) stars before being incorporated in the molecular cloud from which the solar system Click on Term to Read More containing anomalous Xe isotopes (Xe-HL) have been identified in Renazzo. Xe-HL is a mixture of Xe-H (enriched in heavy xenon isotopes) and Xe-L (enriched in light xenon isotopes) (Bekaert et al., 2018 and references therein). Rare presolar silicate grains representing ~18 ppmParts per million (106). Click on Term to Read More, plus rare presolar silicon carbidePresolar interstellar dust grain found in CM and E chondrites; its formula is SiC. Click on Term to Read More grains representing ~55 ppm, have been identified in fine-grained accretionary chondrule rims (Leitner et al., 2012). Refractory inclusionsInclusions found predominantly in carbonaceous chondrites and are rich in refractory elements particularly calcium, aluminum and titanium that in various combinations form minerals such as spinel, melilite, perovskite and hibonite. There are two types of refractory inclusion: • Ca Al-rich inclusions (CAIs) • Amoeboid olivine aggregates (AOAs) Refractory inclusions were Click on Term to Read More in Renazzo are small (generally <1 mm and most <0.5 mm) and scarce, just as in other CR chondrites. They include pristine 16O-rich CAIs that were formed over a period of ~100,000 to 400,000 years in a similar nebular reservoir as those in CV chondrites (Makide et al., 2009). CAIs constitute <1 vol% of CR chondrites and have primarily melilite-rich compositions, while others are grossite- or hibonite-rich, or more rarely, anorthite-rich. Fine-grained aggregates of nebular gas-solid condensation, known as amoeboid olivine aggregatesMillimeter sized, fine-grained inclusions present to a few volume-percent in most carbonaceous chondrites. They can be round but can also be irregularly shaped like an amoeba (thus the name amoeboid). They are forsterite (Mg-rich olivine) and Ca-Al-Ti mineral aggregates. The most characteristic texture of AOAs is an anorthite core (sometimes Click on Term to Read More (AOAs), are minor constituents in Renazzo. These AOAs preserve some of the most primitive relicts of early nebular condensation similar to those present in CAIs, including refractory minerals such as perovskite and spinelMg-Al oxide, MgAl2O4, found in CAIs., and Mn-rich forsterite; primary FeNi-metal blebs also occur in some (Weisberg et al., 2008). Evidence indicates that AOAs formed during a period intermediate between the final stages of Wark-Lovering rim formation on type-A CAIs and the onset of chondrule formation. The occurrence of CAI–chondrule compound objects attests to subsequent remelting of some CAIs with chondrules in an evolved, 16O-depleted solar nebula. Both AOAs and CAIs have similar 26Mg excesses derived from initial 26Al values, and they share an 16O-rich composition likely derived from the same nebular gas reservoir (Weisberg et al., 2004; 2007). Following their formation, AOAs did not experience further equilibration with the cooling nebular vapor. The small component of Al-rich (>10 wt% Al2O3) chondrules are thought to have formed by melting of spinel–anorthite–pyroxene CAI precursor material that was mixed with type-I precursor material (Krot et al., 2006). In contrast to the 16O-poor type-I and -II ferromagnesian chondrules, a significant percentage of Al-rich chondrules exhibit O-isotopic heterogeneity due to inclusion of 16O-rich relict CAI material, and to isotopic exchange processes with an evolving nebular gas. The CR group contains unusually high abundances of FeNi-metal in the form of taeniteLess common than kamacite, both taenite and kamacite are Ni-Fe alloys found in iron meteorites. Taenite, γ-(Fe,Ni), has 27-65 wt% Ni, and forms small crystals that appear as highly reflecting thin ribbons on the etched surface of a meteorite; the name derives from the Greek word for "ribbon." Click on Term to Read More and kamaciteMore common than taenite, both taenite and kamacite are Ni-Fe alloys found in iron meteorites. Kamacite, α-(Fe,Ni), contains 4-7.5 wt% Ni, and forms large body-centered cubic crystals that appear like broad bands or beam-like structures on the etched surface of a meteorite; its name is derived from the Greek word Click on Term to Read More (5–9 vol%), and the metal-bearing sulfides pyrrhotite and pentlandite (1–4 vol%) contribute to this high metal content. FeNi-metal occurs in Renazzo in chondrule interiors, on chondrule rims, and as separate finer grains in the matrix. It has been suggested (Connolly et al., 2001; Zanda et al., 2002) that during the heating event(s) in which chondrules were forming, FeO and other volatiles present in the precursor condensates were evaporated and then recondensed onto the chondrule rims, later diffusing inward. The chondrules that were melted to the highest degree, corresponding to those with the most circular shapes, developed the highest abundance of metal grains on their rims. Because of the evaporation and migration of Fe to the rim metal—the most stable arrangement (factoring in properties such as surface tension and temperature)—and then its incomplete rediffusion back into the interior metal, the metal in the chondrule interiors became enriched in Ni, P, and other siderophile components. Trace element data and Ni–Co correlations support this scenario, although they indicate that certain components of chondrule metal, especially the coreIn the context of planetary formation, the core is the central region of a large differentiated asteroid, planet or moon and made up of denser materials than the surrounding mantle and crust. For example, the cores of the Earth, the terrestrial planets and differentiated asteroids are rich in metallic iron-nickel. Click on Term to Read More grains, did originate through direct, high temperature nebular condensation processes (Schönbeck and Palme, 2003; Ebel et al., 2009). The subsequent introduction of the chondrules to an oxidizing environment may also be responsible for the removal of Fe from the core. In contrast to the argument of Wood (1963) and others for a direct condensation model, Wasson and Rubin (2010) proposed that a nebular fractional crystallizationPhysical or chemical process or action that results in the formation of regularly-shaped, -sized, and -patterned solid forms known as crystals. Click on Term to Read More process, occurring at ~1750K and proceeded by a slow cooling rate, was responsible for producing the observed Ni gradients (core to rim decrease) and granoblastic textures of chondrules. They found that this nebular process, rather than either reduction of FeO or evaporation/recondensation, was more consistent with creating the positive correlation between Co and Ni in surface metal, as well as the negative correlation between olivine Fa content and Ni. The subsequent evaporation of S from the surficial FeS component of the chondrule rim would then increase the interface tension, ultimately leading to surface energy forces influencing the formation of coarse metal globules rather than a potential homogeneous metal film. Still, other components of CR metal are consistent with an origin through high-temperature silicate reduction and metal–silicate equilibrium processes, as evidenced through results of Fe-isotopic mass fractionationFractionation of isotopes or elements that is dependent on their masses. Click on Term to Read More studies. Experimental results by Cohen et al. (2006) demonstrate that type-I chondrule metal is consistent with formation by such a reduction process, and constrains the associated chondrule formation time to ~1 hour. Based on the results of evaporation experiments in a low-pressure furnace, Cohen and Hewins (2004) advanced a model in which the FeNi-metal found in Renazzo and other chondrites was a product of desulfurization of sulfides through volatilation, possibly as FeS liquid was condensing from the solar nebula at high temperature (~1565°C) and high pressure (~1 atm); the heat for this process may have been generated by the passage of a shock waveAbrupt perturbation in the temperature, pressure and density of a solid, liquid or gas, that propagates faster than the speed of sound.. They also argued that the FeNi-metal inclusions present within olivine grains, commonly known as ‘dusty olivine’, are best modeled as having been formed through the reduction of FeO in the presence of carbonElement commonly found in meteorites, it occurs in several structural forms (polymorphs). All polymorphs are shown to the left with * indicating that it been found in meteorites and impact structures: a. diamond*; b. graphite*; c. lonsdalite*; d. buckminsterfullerene* (C60); e. C540; f. C70; g. amorphous carbon; h. carbon nanotube*. Click on Term to Read More (kerogen). ∗ In an effort to establish a definitive history of the FeNi-metal in CR chondrites, Jacquet et al. (2013) conducted trace element analyses of numerous metal grains from nine CR chondrites. These metal grains were present in three configurations—as chondrule ‘interior grains’, as chondrule surface, rim, or ‘margin grains’, and as chondrite matrix or ‘isolated grains’. After examining the geochemical relationships among these different metal grains, a formation scenario for CR type-I chondrules was developed which is consistent with the totality of their findings—some aspects of their scenario are contrary to portions of previous propositions outlined above:
- Precursor grains were produced under conditions of incomplete condensation, inheriting a depleted volatile element abundance, and began to aggregate into chondrules.
- As small interior metal grains were heated and melted, they coalesced to form rounded grains.
- An extended period (>1 day) of high temperatures resulted in equilibration between the interior metal grains and olivine, accompanied by evaporative loss of some Fe from those chondrules which were completely melted (leaving them Ni-enriched); an open-system redoxOxidation and reduction together are called redox (reduction and oxidation) and generally characterized by the transfer of electrons between chemical species, like molecules, atoms or ions, where one species undergoes oxidation, a loss of electrons, while another species undergoes reduction, a gain of electrons. This transfer of electrons between reactants Click on Term to Read More processing is favored by the evidence.
- Continued accretion and heating of fine-grained material onto these early-formed chondrules produced the igneous rims; this period reflects either a single prolonged accretional/heating event, or possibly involves multiple heating events.
- As the cooling rate began to increase, a layer of pyroxene formed at the chondrule periphery, possibly by interaction with a Si-enriched gas, and these newly accreted margin grains coalesced and experienced a lower-degree of melting to form zoned amoeboid-shaped grains.
- Continued accretion of some fine-grained material onto chondrules proceeded.
- If a single localized heating episode was involved, whereas these stages occurred concurrently, then this may be indicative of these chondrules forming in a high-temperature region with a high dust densityMass of an object divided by its volume. Density is a characteristic property of a substance (rock vs. ice, e.g.). Some substances (like gases) are easily compressible and have different densities depending on how much pressure is exerted upon them. The Sun is composed of compressible gases and is much Click on Term to Read More, followed by a sudden onset of cooling upon exiting this region; the temperature curve fitting this scenario is inferred to be inconsistent with that of the often cited shock wavePropagating disturbance which transmits energy from one point to another without physically transporting the oscillating quantity. A wave is characterized by wavelength and frequency. model of chondrule formation.
Sketch of the formation scenario for CR type-I chondrules
Image credit: Jacquet et al., MAPS, vol. 48, #10, p. 1995 (2013)
‘Trace element geochemistryStudy of the chemical composition of Earth and other planets, chemical processes and reactions that govern the composition of rocks and soils, and the cycles of matter and energy that transport Earth's chemical components in time and space. Click on Term to Read More of CR chondrite metal’ (http://dx.doi.org/10.1111/maps.12212) In their studies of primitive chondrites, Kimura et al. (2008) found that the FeNi-metal phases serve as one of the most sensitive indicators of the onset of thermal metamorphism. Their work reveals that primary martensite decomposes to fine-grained plessiteA fine-grained intergrowth of kamacite and taenite that fills in the wedges between wide kamacite and taenite bands in octahedrites. The name derives from the Greek word for "filling." Click on Term to Read More during low degrees of metamorphism as observed in the LL chondriteOrdinary chondrites ("low Fe" / "low metal") with only 1 to 3% free metal. Their olivine is more Fe-rich than in the other ordinary chondrites (Fa27-32), implying that the LL types must have formed under more oxidizing conditions than their H or L cousins. Orthopyroxene compositions are also Fe-the rich Click on Term to Read More Semarkona, but that this has not occurred in the more pristine ungroupedModifying term used to describe meteorites that are mineralogically and/or chemically unique and defy classification into the group or sub-group they most closely resemble. Some examples include Ungrouped Achondrite (achondrite-ung), Ungrouped Chondrite (chondrite-ung), Ungrouped Iron (iron-ung), and Ungrouped Carbonaceous (C-ung). Click on Term to Read More (probably CO-related; Simon and Grossman, 2015) carbonaceous chondrite Acfer 094. Furthermore, they found that metal in and around Semarkona chondrules does not show a solar ratio of Co/Ni like that in Acfer 094, and that low temperature aqueous alteration has occurred in Semarkona as well. In addition, Kimura et al. (2008) included the carbonaceous chondrites of groups CR, CH, CB, and CM as probable 3.00 subtype specimens, notwithstanding the fact that some are currently designated as subtype 2 due to aqueous alteration features. In light of this petrologic typing paradox, they proposed that a separate scale be adopted to describe aqueous alteration which is distinct from the scale currently used for thermal metamorphism.
- chondrule igneous rim material (and later, mesostasisLast material to crystallize/solidify from a melt. Mesostasis can be found in both chondrules, in the matrix around chondrules, and in achondrites as interstitial fine-grained material such as plagioclase, and/or as glass between crystalline minerals. Click on Term to Read More) is altered to phyllosilicates
- matrix S concentration decreases
- clear isotropicSame in all directions. Click on Term to Read More glass becomes altered (e.g., leaching, zoning, hydrationReaction of a substance with water. Click on Term to Read More)
- FeNi-metal grains undergo conversion to magnetite, along with increasing abundances of oxide phases and Fe-carbonate
- Δ17O values tend to increase from ~ –2.6‰ in type 2.8 to ~ –0.4‰ in type 2.0, likely due to exchange with 16O-poor fluids
- abundances of amino acids and O-anomalous presolar grains tend to decrease
According to this scale, Renazzo has been designated petrologic subtype 2.4. Hydrothermal alteration phases are abundant in Renazzo, including serpentineName used for a large group of phyllosilicate minerals with the generalized formula X2-3 Y2 O5 (OH)4. Due to their various structures (meteoritics focuses primarily on (Fe, Mg)3Si2O5(OH)4), serpentine can be used to understand the chemistry and progress of aqueous alteration (hydration) of olivine, amphibole, or pyroxene dating back to Click on Term to Read More, smectite, and certain chlorite group minerals, which suggest an alteration temperature no higher than ~50–150°C; other CR chondrites have compositions more consistent with alteration temperatures as high as ~300°C. This isotopically heavy fluid (D-enrichment higher than that found in comets) has transformed the composition of certain CR samples to a greater degree than others—the CR2.3 chondrite Al Rais represents an isotopically heavy endmember, while the CR2.8 QUE 99177 represents an isotopically light endmember. The variability of D-enrichment within samples has been attributed to parent body processes rather than to accretion of an ice mixture (Bonal et al., 2013). Studies by Abreu and Stanek (2012) demonstrate that the CR chondrites which experienced the highest degrees of aqueous alteration now have the lowest Fe and the highest S content, while those that experienced the highest shock stages accompanied by volatile loss now have the highest Fe and the lowest S contents. The water/rock ratio on the CR parent body was calculated to be as high as 1.157 (Schrader et al., 2010).
Abundant xenolithic dark clasts are present in CR chondrites (~8 vol%), but oxygen isotopic data indicate their precursor is different from the CR parent body; they were likely accreted by the CR parent body during its early formative stage. Oxygen isotopic compositions and mineralogical characteristics of these dark clasts show similarities to type 3 carbonaceous chondrites that were aqueously altered prior to their incorporation into the CR host. Important similarities exist between the CR group and the CI group, suggesting that they both formed in a similar nebular region, but with the CR chondrites undergoing reduction during metamorphism and hydrothermal alteration. Interstellar-sourced organicPertaining to C-containing compounds. Organic compounds can be formed by both biological and non-biological (abiotic) processes. Click on Term to Read More compounds enriched in deuteriumAlso called heavy hydrogen, deuterium is an isotope of hydrogen (D, or 2H) whose nucleus contains one proton and one neutron. As a trace element formed during the nucleosynthesis epoch of the Big Bang, deuterium is an important indicator of the baryon density in the universe. The larger the density, the Click on Term to Read More (D) and heavy nitrogenPrincipal constituent of the Earth’s atmosphere (78.08 vol. % at ground level). Nitrogen is the fifth most abundant element in the universe by atom abundance. Nitrogen comprises only 3.5 vol. % of the atmosphere of Venus and 2.7 vol. % of Mars’s atmosphere. Nitrogen has two isotopes: 14N (99.632 %) and 15N Click on Term to Read More (15N) are present in Renazzo and other CR chondrites, which is considered to reflect accretion within the cold outer region of the protoplanetary disk (Budde et al., 2006 and references therein). These isotopes are associated with carbon (C) contents of between 1.2 and 2.7 wt%. These organic macromolecules are composed of mostly 1–4 ring (up to 15 rings have been identified) aromatic C compounds comprising both oxidizedOxidation and reduction together are called redox (reduction and oxidation) and generally characterized by the transfer of electrons between chemical species, like molecules, atoms or ions, where one species undergoes oxidation, a loss of electrons, while another species undergoes reduction, a gain of electrons. This transfer of electrons between reactants Click on Term to Read More (hydrous alteration) and reducedOxidation and reduction together are called redox (reduction and oxidation) and generally characterized by the transfer of electrons between chemical species, like molecules, atoms or ions, where one species undergoes oxidation, a loss of electrons, while another species undergoes reduction, a gain of electrons. This transfer of electrons between reactants Click on Term to Read More (anhydrous alteration) species. Minimal aqueous processing in Renazzo and other CR carbonaceous chondrites is indicated by the low degree of hydroxylation of toluene to phenols, and by the failure to liberate 15N-rich organic species, as well as by the presence in the insoluble organic material of an inclusion with the largest known alkyl component (Cody and Alexander, 2005). In addition, the organic material maturity parameter, PAI 1, indicates only mild aqueous alteration was involved (Pearson et al., 2006). The low degree of sulfur-based thiophenes is indicative of a low degree of thermal metamorphism. In addition, through organic material maturity parameters (such as MNR), it is demonstrated that Renazzo has experienced the least thermal processing among the CR chondrite members. Floss and Stadermann (2009) identified high abundances of both nanocrystalline and amorphousMaterial without the regular, ordered structure of crystalline solids. Amorphous substances, like glass, lack a definite repeating pattern in their atomic structures (crystallinity). There may be small regions of order, but, overall there is disorder. Click on Term to Read More, O-anomalous, ferromagnesian presolar silicate grains in the mostly unaltered CR2.8 chondrites QUE 99177 and MET 00426 (hydrated to serpentine in the latter; Le Guillou et al., 2013). These presolar grains are thought to have originated in oxygen-rich, low-mass red giantGiant and highly luminous red star in the later stages of stellar evolution after it has left the main sequence. These red stars have a relatively cool surface whose core has burned most of its hydrogen. Red giants lose parts of their atmospheres and thus provide new elements into interstellar Click on Term to Read More and asymptotic giant branch (AGB) starsStars on the Asymptotic Giant Branch, which represents a late stage of stellar evolution that all stars with initial masses < 8 Msun go through. At this late stage of stellar evolution, gas and dust are lifted off the stellar surface by massive winds that transfer material to the interstellar Click on Term to Read More. The silicate/oxide ratios of these presolar grains are higher than those found in the most primitive meteorites analyzed (Acfer 094 and ALHA77307), and are similar to those found in interplanetary dust particlesExtremely small (~10 μm in diameter) particles found on Earth (or collected during high altitude flights) that are probably from outer space. Their small size poses a problem for most common chemical and petrographic analytical techniques and thus research into IDPs is marked by the application of new analytical procedures Click on Term to Read More (IDPs); this attests to the pristine nature of these CR chondrites, and may indicate the actual protosolar cloud abundances of presolar silicate and oxide grains. These presolar grains also contain primitive C of interstellar origin and have a high amino acidA small molecule containing and amine group (–NH2) at one end and a carboxylic acid group (–COOH) at the other. They link up with various other amino acids to form proteins. Because they are used to build proteins, most of the human body consists of amino acids with their abundance second Click on Term to Read More content. It appears likely that the high degree of aqueous alteration experienced by other CR chondrites is the reason they lack similar presolar silicate grains. In the CR2.8 GRV 021710, an abundance of 174 (±30) ppm O-anomalous grains, 203 (±36) ppm C-anomalous grains, 165 (±29) ppm presolar silicate grains, 9 (±6) ppm presolar oxide grains, 135 (±35) ppm SiC grains, and 50 (±12) ppm carbonaceous grains were calculated to be present (Zhao et al., 2011). It was discovered that some Antarctic CR chondrites contain the highest amino acid abundances measured in any meteorite group. These are extraterrestrial amino acids as evidenced by their enrichment of 13C, and they have C-isotope values similar to those present in CM2 chondrites, possibly indicating a common presolar precursor. It is thought that final synthesis of certain amino acids, such as the α-amino acids alanine, glycine, isovaline, and α-aminoisobutyric acid, took place on the parent asteroid through aqueous alteration of existing presolar carbonyl precursors (Martins et al., 2007). Renazzo has a relatively low abundance of amino acids, probably due to degradation through higher degrees of aqueous alteration. Using Raman spectroscopyTechnique of splitting electromagnetic radiation (light) into its constituent wavelengths (a spectrum), in much the same way as a prism splits light into a rainbow of colors. Spectra are not smooth but punctuated by 'lines' of absorption or emission caused by interaction with matter. The energy levels of electrons in, researchers have demonstrated that the degree of structural order of the organic matter in Renazzo and other unequilibrated ordinary chondrites is correlated with their petrologic type (Quirico et al., 2003). They also found that the abundances of D and 15N were also correlated. From these data they determined that Renazzo contains the most pristine organic matter among all chondrites, and that LL3.01 Semarkona, which was identified as being slightly less primitive than Renazzo, is by far the most pristine among the ordinary chondriteWork in Progress Ordinary chondrites (OCs) are the largest meteorite clan, comprising approximately 87% of the global collection and 78% of all falls (Meteoritical Society database 2018)1. Meteorites & the Early Solar System: page 581 section 6.1 OC of type 5 or 6 with an apparent shock stage of S1, Click on Term to Read More groups. In addition, a study of FeNi-metal and sulfide composition and texture by Kimura et al. (2006) has revealed that the anomalous carbonaceous chondrite Acfer 094 experienced even less metamorphism than Semarkona, and is consistent with the lowest petrologic type assignment of 3.00. One CR chondrite with a very high degree of hydration, GRO 95577, was classified by Weisberg and Huber (2007) as the first CR1 chondrite (CR2.0 after Harju et al., 2011). Although the chondrules have been completely replaced by phyllosilicate layers, magnetite, and various sulfides, their textural integrity has been preserved and they are still discernible as type-I. Similarly, all of the metal has been replaced by magnetite, and there is a prevalence of sulfides and carbonates where matrix and dark inclusions had previously been located. Alteration processes involving hydrothermal fluids probably occurred at temperatures of ~300°C. Also of note is a meteorite previously classified as C3-ungrouped, Sah 00182, which shares many similarities with the CR chondrite group (Weisberg, 2001). In addition to having similar chondrule textures, most chondrules in both Sah 00182 and other CR members are Mg-rich type-I. Metal within chondrules has a similar Ni content (4.7–6.6 wt%) and Co/Ni ratio. Although Sah 00182 lacks hydrous phyllosilicates consistent with a petrologic grade of 3, it is nevertheless petrographically similar to the CR chondrites given their degree of aqueous alteration. However, the O-isotopic ratios of Sah 00182 plot within the field of the CV chondrites, and therefore Sah 00182 remains an ungrouped carbonaceous chondrite which has retained a pristine nebular signature within its components (chondrules, FeNi-metal, CAIs, AOAs), and it has experienced very little thermal metamorphism. In addition, a few meteorites have alteration histories that may be consistent with a CR3 classification, including Acfer 324 (Sipiera and Cole, 2004). Although recognizing the existence of significant petrological differences among them, Weisberg et al. (1995) proposed to include the CB, CH, and CR chondrite groups within a clan hierarchy based on similarities in mineralogical, bulk chemical, and O- and N-isotopic characteristics. In a contrary opinion, Makide et al. (2009) argue that the CR chondrite group should not be included with the CB and CH groups because of its dissimilarities in chondrule texture, metal abundance, matrix abundance, CAI composition and isotopic systematics, and component history (pristine nebula vs. impact vapor plume). The ungrouped C3 chondrite LEW 85332 (Rubin and Kallemeyn, 1990) is considered to be the closest match to the likely precursor material of the CR clan with regard to its isotopic and chemical properties (Clayton and Mayeda, 1999). Notably, it has been proposed by Alexander and Bowden (2018, #6063) that LEW 85332 and the CR2 chondrite MIL 090001 represent a duo from a unique parent body. From comparisons made between the reflectance spectrum of asteroid 2 Pallas and that of the carbonaceous chondrite groups, it was shown that a close similarity exists to Renazzo. Compared to all other meteorite groups, both CR chondrites and IIC irons have significant δ183W excesses, have elevated δ15N, and share similar Mo isotope systematics, and therefore a genetic link is inferred (Kruijer et al., 2017; Budde et al., 2018). It has been proposed by many investigators that a large (~400 km diameter) differentiated CR parent body formed in the early history of the solar system and subsequently experienced a collisional disruption. For more information pertaining to this scenario, see the LPSC abstract ‘Primitive’ and igneous achondrites related to the large and differentiated CR parent body by Bunch et al. (2005), and the MetSoc abstract Tafassasset and Primitive Achondrites: Records of Planetary Differentiation by Nehru et al. (2014). The diagram below includes numerous meteorites that plot within the CR chondrite field and potentially represent daughter components of a single large disaggregated body.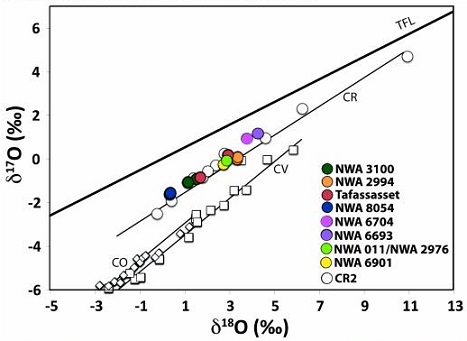
Diagram credit: Sanborn et al., 45th LPSC, #2032 (2014) Huyskens et al. (2019) derived and compiled chronological data from multiple dating systems for four different achondriteAn achondrite is a type of stony meteorite whose precursor was of chondritic origin and experienced metamorphic and igneous processes. They have a planetary or differentiated asteroidal origin where the chondritic parent body reached a sufficient size that through heating due to radioactive decay of 26Al (aluminum isotope) and gravitational Click on Term to Read More parent bodies that accreted in the CR reservoir, comprising the pairing groups of NWA 011/2976/4587, NWA 6704/6693/10132, Tafassasset/NWA 3100, and NWA 6962/7680. They determined that each of these parent bodies accreted and differentiated early in Solar System history and over a relatively short timespan ~4.5637 to 4.5624 b.y. ago. Each of these CR-like objects have Cr- and Ti-isotopic compositions that when coupled to the O-isotopic compositions plot in distinct locations (see diagrams below). Notably, the CR2 chondrite Renazzo plots nearest to NWA 6962/7680 in O–Cr space, but no comparable Ti isotope data is yet available. 17O vs. ε54Cr and ε50Ti for CR Carbonaceous Achondrites
click on photo for a magnified view Diagrams credit: Huyskens et al., 50th LPSC, #2736 (2019)
The brecciated nature of Renazzo is also manifest as heterogeneity in its porosity, which ranges from 3.7% to 18.2% (Macke et al., 2011). The specimen of Renazzo pictured above is a 1.1 g partial end section, showing both the interior with armored chondrules and the exterior with fresh fusion crustMelted exterior of a meteorite that forms when it passes through Earth’s atmosphere. Friction with the air will raise a meteorite’s surface temperature upwards of 4800 K (8180 °F) and will melt (ablate) the surface minerals and flow backwards over the surface as shown in the Lafayette meteorite photograph below. Click on Term to Read More.