CM2.5
(HydrationReaction of a substance with water. Click on Term to Read More subtype 1.6)
Fell September 28, 1969
36° 37′ S., 145° 12′ E. On a Sunday morning around 10:45, a fireballA fireball is another term for a very bright meteor, generally brighter than magnitude -4, which is about the same magnitude of the planet Venus as seen in the morning or evening sky. A bolide is a special type of fireball which explodes in a bright terminal flash at its end, often with visible fragmentation. Click on Term to Read More exploded with loud detonations and hissing noises. Hundreds of stones rained down over Victoria, Australia covering 5 square miles, permeating the town with the odor of alcohol. Over 700 charcoal-colored stones with a total weight of over 100 kg were collected, the largest mass weighing 7 kg.
Degree of Alteration | |
---|---|
Stage-I | unaltered or least altered |
Stage-II | weakly altered (affecting mesostasisLast material to crystallize/solidify from a melt. Mesostasis can be found in both chondrules, in the matrix around chondrules, and in achondrites as interstitial fine-grained material such as plagioclase, and/or as glass between crystalline minerals. Click on Term to Read More) |
Stage-III | moderately altered (affecting Ca-poor pyroxeneA class of silicate (SiO3) minerals that form a solid solution between iron and magnesium and can contain up to 50% calcium. Pyroxenes are important rock forming minerals and critical to understanding igneous processes. For more detailed information, please read the Pyroxene Group article found in the Meteoritics & Classification category. Click on Term to Read More) |
Stage-IV | intensely altered (affecting olivineGroup of silicate minerals, (Mg,Fe)2SiO4, with the compositional endpoints of forsterite (Mg2SiO4) and fayalite (Fe2SiO4). Olivine is commonly found in all chondrites within both the matrix and chondrules, achondrites including most primitive achondrites and some evolved achondrites, in pallasites as large yellow-green crystals (brown when terrestrialized), in the silicate portion Click on Term to Read More) |
Utilizing petrography and mineralogy, Browning et al. (1996) observed several features correlated to an increase in hydration, among which was a progression from Fe-rich serpentineName used for a large group of phyllosilicate minerals with the generalized formula X2-3 Y2 O5 (OH)4. Due to their various structures (meteoritics focuses primarily on (Fe, Mg)3Si2O5(OH)4), serpentine can be used to understand the chemistry and progress of aqueous alteration (hydration) of olivine, amphibole, or pyroxene dating back to Click on Term to Read More to Mg-rich serpentine. Based on these indicators they arranged a set of CM chondriteClass of carbonaceous chondrites named after the Mighei meteorite that fell in Ukraine in 1889. They represent samples of incompletely serpentinized primitive asteroids and have experience extremely complex histories. CM meteorites are generally petrologic level type 2 though a few examples of CM1 and CM1/2 also exist. Compared to CI Click on Term to Read More falls in a sequence of increasing relative aqueous alteration as follows: Murchison ≤ Bells < Pollen ≤ Murray < Nogoya < Mighei < Cold Bokkeveld Results of a petrological, geochemical, and reflectance spectral study (diversity in the 3 µm band center) involving a set of CM chondrites, conducted under dry and vacuumSpace entirely devoid of matter (called also, by way of distinction, absolute vacuum). In a more general sense, a space, as the interior of a closed vessel, which has been evacuated to a high degree by a pump or other artificial means. conditions, has led Takir et al. (2013) to establish three spectral groups corresponding to an increasing state of hydration, and which could be applied to the interpretation of spectroscopic observations of asteroids:
- Group 1 (least aqueously altered) phyllosilicatesClass of hydroxyl-bearing silicate minerals with a sheet-like structure. They result from aqueous alteration are dominantly serpentine and smectite in meteorites; found in the matrixes of carbonaceous chondrites. Phyllosilicates consist of repeating sequences of sheets of linked tetrahedra (T) and sheets of linked octahedra (O). The T sheet consists of Click on Term to Read More composed of Fe-serpentine (cronstedtite) corresponding to subtypes 2.6–2.3
- Group 2 (intermediate aqueous alteration with intermediate mineralogical phases)
- Group 3 (most aqueously altered) phyllosilicates composed of Mg-serpentine (antigoriteName used for a large group of phyllosilicate minerals with the generalized formula X2-3 Y2 O5 (OH)4. Due to their various structures (meteoritics focuses primarily on (Fe, Mg)3Si2O5(OH)4), serpentine can be used to understand the chemistry and progress of aqueous alteration (hydration) of olivine, amphibole, or pyroxene dating back to Click on Term to Read More) corresponding to subtypes 2.2–2.1
Building upon and strengthening the basis for a unique scale reflecting the degree of aqueous alteration, Rubin et al. (2005, 2007) proposed a new alteration sequence for CM chondrites. Based on eight major diagnostic parameters of progressive alteration, they classified Murchison as a petrologic typeMeasure of the degree of aqueous alteration (Types 1 and 2) and thermal metamorphism (Types 3-6) experienced by a chondritic meteorite. Type 3 chondrites are further subdivided into 3.0 through 3.9 subtypes. 2.5, which, along with the other CM chondrites, was derived from a hypothetical precursor lithology having a petrologic type 3.0, broadly similar to the anhydrous, ungroupedModifying term used to describe meteorites that are mineralogically and/or chemically unique and defy classification into the group or sub-group they most closely resemble. Some examples include Ungrouped Achondrite (achondrite-ung), Ungrouped Chondrite (chondrite-ung), Ungrouped Iron (iron-ung), and Ungrouped Carbonaceous (C-ung). Click on Term to Read More (probably CO-related; Simon and Grossman, 2015), type-3.0 Acfer 094, or perhaps the CO3.0 ALHA77307. Following are the major parameters developed by Rubin et al. (2007) for estimating the degree of alteration of the CM chondrites:
early to intermediate stage alteration processes:- hydration of fine-grained matrices to form phyllosilicates, which gradually consists of Mg-rich serpentines
- conversion of primary chondruleRoughly spherical aggregate of coarse crystals formed from the rapid cooling and solidification of a melt at ~1400 ° C. Large numbers of chondrules are found in all chondrites except for the CI group of carbonaceous chondrites. Chondrules are typically 0.5-2 mm in diameter and are usually composed of olivine Click on Term to Read More glass to phyllosilicate
- production of large PCP (tochilinite–cronstedtite intergrowths [TCI]) clumps
processes occurring throughout the alteration sequence:
- oxidationOxidation and reduction together are called redox (reduction and oxidation) and generally characterized by the transfer of electrons between chemical species, like molecules, atoms or ions, where one species undergoes oxidation, a loss of electrons, while another species undergoes reduction, a gain of electrons. This transfer of electrons between reactants Click on Term to Read More of FeNi-metal
- alteration of chondrule silicateThe most abundant group of minerals in Earth's crust, the structure of silicates are dominated by the silica tetrahedron, SiO44-, with metal ions occurring between tetrahedra). The mesodesmic bonds of the silicon tetrahedron allow extensive polymerization and silicates are classified according to the amount of linking that occurs between the phenocrysts
- compositional and size changes of TCI (e.g., depletion in S and ‘FeO’ [FeO in phyllosilicates, Fe+3 in cronstedtite, and Fe+2 in sulfide]; SiO2, TiO2, Cr2O3, MnO, and CaO enrichment; decrease in size)
- formation of increasingly complex carbonates (e.g., calcite => dolomite)
- compositional changes in sulfides
Howard et al. (2015) established an aqueous alteration scale for the CM chondrites (as well as CR and C2-ungrouped chondrites) based on the phyllosilicate fraction (total phyllosilicate/total anhydrous silicate + total phyllosilicate). Through modal measurments utilizing Position Sensitive DetectorAny device used to sense the passage of a particle or photon (x-ray, γ-ray, etc.). X-rays can be detected using sealed-gas proportional, gas-flow proportional detectors, and Li-drifted Si semiconductor detectors. A Li-drifted Ge detector is used to count γ-rays in the laboratory. Click on Term to Read More X-ray DiffractionAnalytical technique used to determine the structures of crystalline solids. A monochromatic beam of X-rays (usually Cu-Kα) is diffracted off repeating planes of atoms in crystalline samples to produce a diffraction pattern. Through analysis of the diffraction pattern, atomic structures can often be determined. (PSD-XRD), and defining the sub-types in increments of 5% of the abundance of phyllosilicate, they found that CM chondrites fallMeteorite seen to fall. Such meteorites are usually collected soon after falling and are not affected by terrestrial weathering (Weathering = 0). Beginning in 2014 (date needs confirmation), the NomComm adopted the use of the terms "probable fall" and "confirmed fall" to provide better insight into the meteorite's history. If Click on Term to Read More in the range of 1.7–1.2 in their classification scheme (see example below). However, King et al. (2015) reasoned that since alteration might continue even after all silicates have been hydrated, this scheme could be difficult to extend to CI chondrites in which members reflect similar phyllosilicate abundances but show variable degrees of alteration in other features. type 3.0: phyllosilicate fraction of <0.05
⇓
type 1.0: phyllosilicate fraction of >0.95
(Rubin CM or Harju CR classification × 0.96) – 0.81 = Howard classification In their study, de Leuw et al. (2009, 2010) found that CM chondrites which have lower petrographic subtypes experienced longer durations of aqueous alteration. Analyzing carbonates in CM chondrites, they determined that correlations in the Mn–Cr systematics were indicative of in situ decay of 53Mn during carbonateMineral or compound containing carbon and oxygen (i.e. calcium carbonate, CaCO3, calcite). Click on Term to Read More formation. They were able to utilize the correlation between the degree of aqueous alteration and the age of carbonate formation to arrive at a duration for such alteration of at least 4 m.y. It was shown that the carbonates that are the least altered are the oldest and vice versa. It was also concluded that FeCO3 can be used as a taxonomic parameter. The least-altered CM chondrites (petrologic type ≥2.4) have more abundant FeCO3 contents than those that are more-altered (petrologic type ≤2.4). Features present in the FeNi-metal and sulfides in CM chondrites have been identified which reflect the heating stage as follows (Kimura et al., 2009; Nakamura, 2005):
Stage I | Stage II | Stage III | Stage IV | |
---|---|---|---|---|
Temperature | <300°C | 300–500°C | 500–750°C | >750°C |
Major Features | ||||
Examples |
Kimura et al. (2011) revised this classification scheme by assigning a heating stage category A–C to CM chondrites. Category A (unheated) corresponds to heating stage I above. Category B (300°C to 750°C) corresponds to heating stage II and III above. Category C (>500°C to >750°C) corresponds to heating stage III and IV above. The presence of primordial metal in the form of martensite attests to a low degree of secondary thermal alteration following aqueous alteration in the case of Murchison. The placement of Murchison in Category A is a reflection of the metal composition (Ni content) and the sulfide texture (abundance of pentlandite blebs in pyrrhotite) of this meteoriteWork in progress. A solid natural object reaching a planet’s surface from interplanetary space. Solid portion of a meteoroid that survives its fall to Earth, or some other body. Meteorites are classified as stony meteorites, iron meteorites, and stony-iron meteorites. These groups are further divided according to their mineralogy and Click on Term to Read More.
Murchison contains sparse chondrules with diameters between 0.1 mm and 0.5 mm, composed of individual phenocrysts of olivine and pyroxene. Porphyritic chondrules contain some FeNi-metal grains. The chondrule mesostasis has been altered to phyllosilicates—Fe- and Mg-serpentines. The black matrixFine grained primary and silicate-rich material in chondrites that surrounds chondrules, refractory inclusions (like CAIs), breccia clasts and other constituents. Click on Term to Read More constitutes ~48 vol% of the meteorite and is similar to that of the CI matrix, but contains less magnetiteFe oxide, Fe2+Fe3+2O4, containing oxidized iron (Fe3+) found in the matrix of carbonaceous chondrites and as diagnostic component in CK chondrites. In CK chondrites, magnetite is typically chromian, containing several wt. % Cr2O3. Click on Term to Read More. Chondrule fragments and olivine crystals are abundant in the Murchison matrix, while the CAISub-millimeter to centimeter-sized amorphous objects found typically in carbonaceous chondrites and ranging in color from white to greyish white and even light pink. CAIs have occasionally been found in ordinary chondrites, such as the L3.00 chondrite, NWA 8276 (Sara Russell, 2016). CAIs are also known as refractory inclusions since they Click on Term to Read More content is low. Isotopic research indicates that the matrix and chondrules of pristine carbonaceous chondrites probably condensed during the same heating event (Nyquist et al., 2009). Carbonate grains that formed in situ under low temperature conditions of 0–25°C are present in amounts of a few vol%, which consist predominantly of calcite/aragonite with minor amounts of dolomite, along with low abundances of sulfides. Interestingly, newly identified refractory inclusionsInclusions found predominantly in carbonaceous chondrites and are rich in refractory elements particularly calcium, aluminum and titanium that in various combinations form minerals such as spinel, melilite, perovskite and hibonite. There are two types of refractory inclusion: • Ca Al-rich inclusions (CAIs) • Amoeboid olivine aggregates (AOAs) Refractory inclusions were Click on Term to Read More in Murchison composed primarily of hiboniteRefractory mineral, Ca-aluminate (CaAl12O19) that occurs in terrestrial metamorphic rocks and in CAIs of many chondrites. Meteoritic hibonite tends to be blue as seen in the meteorite Isheyevo (Ch/CB). Hibonite is one of the most refractory minerals found in primitive meteorites. Click on Term to Read More represent some of the earliest condensed solids or residues from the early, hot, solar nebulaThe primitive gas and dust cloud around the Sun from which planetary materials formed. (Liu et al., 2009). These refractory grains comprise platy crystals (PLACs), spinel–hibonite spherules (SHIBs), and blue aggregates (BAGs). PLACs lack resolvable 26Mg-excesses and were formed within a timespan of ~100,000 years in an 16O-enriched, heterogeneous region (based on anomalous δ48Ca and δ50Ti isotopic signatures) prior to incorporation and mixing of short-lived nuclides such as 26Al into the solar nebulaAn immense interstellar, diffuse cloud of gas and dust from which a central star and surrounding planets and planetesimals condense and accrete. The properties of nebulae vary enormously and depend on their composition as well as the environment in which they are situated. Emission nebula are powered by young, massive Click on Term to Read More through injection of interstellar dustGrains of carbon and silicate ~0.1-1.0 mm in size. Dust grains are a major component of the interstellar medium. Dust blocks visible light causing interstellar extinction and scatters incident starlight, particularly blue light (which has a wavelength comparable to the dust grain's size), causing reddening. Cooling of interstellar gas and Click on Term to Read More. Both PLAC and BAG formation occurred hundreds of thousands of years prior to the formation of CV CAIsSub-millimeter to centimeter-sized amorphous objects found typically in carbonaceous chondrites and ranging in color from white to greyish white and even light pink. CAIs have occasionally been found in ordinary chondrites, such as the L3.00 chondrite, NWA 8276 (Sara Russell, 2016). CAIs are also known as refractory inclusions since they Click on Term to Read More. In contrast, SHIBs formed later by condensation of precursor material in a lower temperature environment than that of PLACs. They record in situ 26Al decay and ‘canonical’ initial levels of 26Al/27Al, and they are considered to have formed 100,000–300,000 years after the formation of CV CAIs. Some hibonite grains in Murchison have experienced Rayleigh fractionationConcentration or separation of one mineral, element, or isotope from an initially homogeneous system. Fractionation can occur as a mass-dependent or mass-independent process. Click on Term to Read More through distillation/evaporation, while some also exhibit nuclear anomalies showing similarities to the FUN group of CAIs studied in CV3 Allende. X-ray diffraction techniques and Mössbauer spectroscopyTechnique of splitting electromagnetic radiation (light) into its constituent wavelengths (a spectrum), in much the same way as a prism splits light into a rainbow of colors. Spectra are not smooth but punctuated by 'lines' of absorption or emission caused by interaction with matter. The energy levels of electrons in have been used by Bland et al. (2004) to determine the modal mineralogy of several carbonaceous chondrites including Murchison. They were also able to quantify the compositional range of the olivine phases. In addition, the grain densityMass of an object divided by its volume. Density is a characteristic property of a substance (rock vs. ice, e.g.). Some substances (like gases) are easily compressible and have different densities depending on how much pressure is exerted upon them. The Sun is composed of compressible gases and is much Click on Term to Read More can be readily estimated from the mode data, and therefore, in combination with the calculated bulk density, the porosityThe volume percentage of a rock that consists of void space. Vesicular porosity is a type of porosity resulting from the presence of vesicles, or gas bubbles, in igneous rock such as the pumice presented here. Vesicular porosity is very rare in meteorites and is often associated with slag, one Click on Term to Read More can be determined. The modal mineralogy (vol%) and other physical properties of Murchison were determined to be as follows:- Olivine
- Fo100 ——————– 6.8
- Fo80 ——————— 1.9
- Fo50 ——————— 1.6
- Clinoenstatite (En98) ——— 1.9
- Pyrrhotite ———————- 1.8
- Pentlandite ——————— 0.3
- Magnetite ———————– 0.2
- Serpentine ——————— 26.2
- Calcite ————————— 1.2
- Tochilinite/Cronstedtite —- 58.1
- TOTAL ———————– 100.0
- grain density = 2.92 g/cm³
- bulk density = 2.20 g/cm³
- porosity = 24.7 vol%
A more technologically advanced determination of the Murchison modal (vol%) mineralogy was conducted by Howard et al. (2009) with results as follows:
- Olivine —————————15.0
- Clinoenstatite (En98) ———- 8.3
- Pyrrhotite ———————– 1.2
- Pentlandite ——————— 0.65
- Magnetite ———————– 1.1
- Serpentine ——————— 22.0
- Calcite ————————— 1.2
- Cronstedtite —————— 50.0
- TOTAL ———————— 99.45
Dark, fine-grained (<1 µm) material (DFM) which forms opaque mantles surrounding chondrules, refractory inclusions, and matrix silicates, are believed by some to be accretionary features from solar nebula dust reservoirs unrelated to the silicate cores on which they are found. However, results of an investigation by Trigo-Rodriguez et al. (2006) of the variable porosities within these dark rims indicate that they were formed on the parent body through impact-compaction of fine-grained, porous matrix material, followed by aqueous alteration and the deposition within the mantleMain silicate-rich zone within a planet between the crust and metallic core. The mantle accounts for 82% of Earth's volume and is composed of silicate minerals rich in Mg. The temperature of the mantle can be as high as 3,700 °C. Heat generated in the core causes convection currents in Click on Term to Read More of what was historically termed ‘poorly characterized phases’ (PCP). This phase has now been determined to be tochilinite–cronstedtite intergrowths (TCI). The TCI are present in two distinct forms as described by Pignatelli et al. (2016) in the least altered CM chondriteChondrites are the most common meteorites accounting for ~84% of falls. Chondrites are comprised mostly of Fe- and Mg-bearing silicate minerals (found in both chondrules and fine grained matrix), reduced Fe/Ni metal (found in various states like large blebs, small grains and/or even chondrule rims), and various refractory inclusions (such Click on Term to Read More Paris:
- type-I: rounded- or irregular-shaped secondary alteration phase composed primarily of sulfur-rich tochilinite with lesser abundance of cronstedtite and magnetite; usually observed as zoned rims around precursor FeNi-metal beads within chondrules, in which cronstedtite increases in proportion to tochilinite progressing outwards from the rim.
- type-II: round-, anhedral-, or euhedral-shaped secondary alteration phase located within the matrix, constituting two concentric zones of variable thickness; the outer zone is composed of variable proportions of tochilinite and cronstedtite, while the inner zone is composed of a porous, fibrous-textured, Fe-hydroxide mineralInorganic substance that is (1) naturally occurring (but does not have a biologic or man-made origin) and formed by physical (not biological) forces with a (2) defined chemical composition of limited variation, has a (3) distinctive set of of physical properties including being a solid, and has a (4) homogeneous Click on Term to Read More amakinite.
Type-I TCI formed when precursor kamacite particles reacted with ionized, S-bearing, alkaline water at low temperatures of ~50–100°C under reducingOxidation and reduction together are called redox (reduction and oxidation) and generally characterized by the transfer of electrons between chemical species, like molecules, atoms or ions, where one species undergoes oxidation, a loss of electrons, while another species undergoes reduction, a gain of electrons. This transfer of electrons between reactants Click on Term to Read More conditions involving a stable temperature and pressure (Peng et al., 2007). Type-II TCI formed as a complex secondary alteration phase by a step-wise dissolution/reprecipitation process through interaction with an evolving fluid (high-S ⇒ low-S + high-Si ⇒ low-S + low-Si), ultimately replacing olivine, and to a lesser extent, pyroxene grains, in a localized and/or transient, micro-scale aqueous environment (Pignatelli et al., 2016).
Unlike the results of previous studies, it was demonstrated that the DFM is not confined to rims around discrete objects, but instead, can form indistinct boundaries blending seamlessly from the objects into the matrix. Moreover, the dark, fine-grained material can be found extending beyond its associated object, comprising isolated patches within matrix space, forming single rims around multiple objects, and surrounding post-aqueous altertion phases. Many smaller discrete objects have no mantles at all, inconsistent with a common nebular origin. Where DFM composes mantles around discrete objects, it forms a layered structure in which the lowest porosities adjacent to the enclosed objects reflect a high degree of compaction. Because agglomerationProcess of collecting in a jumbled cluster. In relation to meteorites, agglomeration refers to the early accretion of chondrules, refractory inclusions and silicate matrix material to form chondritic clusters. Click on Term to Read More modeling shows that such a high degree of compaction is not attainable through nebular processes, the investigators argue that this provides supporting evidence for a parent body origin for DFM mantling through multiple impact-compaction events. Notably, a microfabric consisting of foliations in some dark inclusions, as well as the preferred orientation of chondrules and matrix phyllosilicates, is likely the result of impact-induced deformation (Lindgren et al., 2012). In a study of deformed chondrules in Murchison using X-ray computed tomography, Hanna et al. (2014) observed both foliation (planar alignment) and lineation (much longer in one dimension than in the other two) fabrics produced by impact stress. Utilizing a combination of high-resolution X-ray computed tomography and 3-D software to study fine-grained rims on CM chondrules, Hanna and Ketcham (2015) found that a significant positive correlation exists between rim thickness and the size of the enclosed chondrule; this result supports the theory that the rims accreted in the solar nebula rather than formation through parent body processes. Following the agglomeration and impact-induced compaction of the various components in Murchison, the material experienced high degrees of aqueous alteration at temperatures of 20–35°C within a zone ~100–250 m thick at a depth of 1–1.8 km (Guo and Eiler, 2007; Hanna et al., 2014). Studies of CM chondrite REEOften abbreviated as “REE”, these 16 elements include (preceded by their atomic numbers): 21 scandium (Sc), 39 Yttrium (Y) and the 14 elements that comprise the lanthanides excluding 61 Promethium, an extremely rare and radioactive element. These elements show closely related geochemical behaviors associated with their filled 4f atomic orbital. Click on Term to Read More patterns indicate the water had a pH of 6–8 during the alteration process (Inoue et al., 2009). Low-temperature reactions involving thin fluid films promoted precipitation–dissolution processes in which Fe- and S-bearing phyllosilicates replaced host minerals. Nanotubes have been identified in both Murchison and Mighei (Zega et al., 2004) which are believed to have condensed from low-temperature, S-rich, aqueous solutions. They are composed of a new serpentine phase intermediate between cronstedtite and chrysotileName used for a large group of phyllosilicate minerals with the generalized formula X2-3 Y2 O5 (OH)4. Due to their various structures (meteoritics focuses primarily on (Fe, Mg)3Si2O5(OH)4), serpentine can be used to understand the chemistry and progress of aqueous alteration (hydration) of olivine, amphibole, or pyroxene dating back to Click on Term to Read More. It was found by Rubin (2012) that the CM chondrites composed of higher-porosity material were affected by higher degrees of shock deformation and fracturing and exhibit stronger petrofabrics, features which facilitated a subsequent commensurate higher degree of aqueous alteration, i.e., lower petrologic subtype. Contrariwise, probably due to their lower porosities, lower matrix abundances, and less bulk water content, the CR-group chondrites do not exhibit a similar correlation between shock features and degree of aqueous alteration (Rubin and Harju, 2012). As shown above, Rubin et al. (2007) consider Murchison to be one of the least altered CM chondrites, and it can be placed within an aqueous alteration sequence with other CM members from most to least aqueously altered as follows: MET 01070 [2.0], QUE 93005 [2.1], Cold Bokkeveld [2.2], QUE 99355 [2.3], Y-791198 [2.4], Murray [2.4/2.5], Murchison [2.5], and QUE 97990 [2.6]. The CM chondrite Paris is a newly discovered representative reflecting a lower degree of aqueous alteration and mild thermal metamorphism. It contains an abundance of well-preserved metal with little magnetite, and has large unaltered zones and less matrix component than other CM members (Zanda et al., 2010). While initially having a CI-like composition, the matrix of CM chondrites reflect a S/Si ratio that decreases as aqueous alteration increases. This pattern demonstrates an independent accretionAccumulation of smaller objects into progressively larger bodies in the solar nebula leading to the eventual formation of asteroids, planetesimals and planets. The earliest accretion of the smallest particles was due to Van der Waals and electromagnetic forces. Further accretion continued by relatively low-velocity collisions of smaller bodies in the Click on Term to Read More within diverse regions of the protosolar disk and a subsequent exchange between two components—a high-temperature fraction and a CI-like fraction—rather than accept the pre-accretion complementarity model involving these two components (Zanda et al., 2011). The unaltered precursor material of the CM group is considered to be type CM3.0. Although Paris does contain some phyllosilicates, most of its features, such as a high content of FeS in PCPs and a high chromium oxide content, indicate a tentative petrologic assignment for hydrothermal alteration of type 2.7/2.8, and a petrologic assignment for thermal metamorphism of A/B (Blanchard et al., 2011). A new investigation of Paris by Rubin (2015) revealed that some regions are even less altered and should be classified as type 2.9. This assignment is similar to that of the ungrouped (probably CO-related; Simon and Grossman, 2015) Acfer 094 and the CO3.0 chondrite ALHA77307. Paris exhibits many intermediate characteristics to the CO chondriteMeteorite class named after the Ornans meteorite that fell in France in 1868, are related in chemistry and composition to the CV chondrites and may, with them, represent a distinct clan of carbonaceous chondrites that formed in the same region of the early solar system. However, COs are usually blacker Click on Term to Read More group (Bourot-Denise et al, 2010). Interestingly, Paris shows signs of pre-terrestrial magnetism, possibly internally generated, attesting to a former convecting metallic coreIn the context of planetary formation, the core is the central region of a large differentiated asteroid, planet or moon and made up of denser materials than the surrounding mantle and crust. For example, the cores of the Earth, the terrestrial planets and differentiated asteroids are rich in metallic iron-nickel. Click on Term to Read More (Cournede et al., 2011). Based on advanced analytical techniques in which the modal mineralogy of a suite of CM chondrites (Mighei, Nogoya, Murchison, Murray, and Cold Bokkeveld) was quantified to a high degree of accuracy, Howard et al. (2009) more accurately resolved the degree of aqueous alteration experienced by these CM chondrites based on their total phyllosilicate abundances, measuring Mg-serpentine + Fe-serpentine (cronstedtite); Fe-rich serpentine transitions to Mg-rich serpentine as aqueous alteration progresses. The investigators found that a narrow range exists in the modal abundances of most CM chondrites with regard to phyllosilicates, anhydrous silicates, and other phases. They showed that the inverse relationship which exists between the anhydrous silicates and the phyllosilicates is reliable evidence that the latter formed from the former through aqueous alteration processes. In addition, the inverse relationship apparent between the abundance of Mg-serpentine and Fe-serpentine (cronstedtite) supports an aqueous alteration process as well; cronstedtite loses Fe and recrystallizes to form Mg-serpentine in the presence of water. At the same time, since this alteration process is controlled by variable abundances of anhydrous silicates within different CM samples, the initial composition of each CM chondrite may have been variable, rendering it impossible to determine the actual extent of aqueous alteration by this method. In light of the fact that accurate measurements of phyllosilicate abundances among the CM samples have now been obtained by Howard et al. (2009), and that these abundances have been ascertained to be the same within a small range (73–79%), it may be inferred that each CM chondrite has experienced an equal degree of aqueous alteration, and the subtypes proposed by Rubin et al. (2007) (as shown above) are not accurately defined. As a result, the team argues that it is not necessary to resolve the subtype of the CM group further than CM2. Be that as it may, Howard and Alexander (2013) have proceded to establish an aqueous alteration classification scale (hydration scale) distinct from the thermal metamorphicRocks that have recrystallized in a solid state due to changes in temperature, pressure, and chemical environment. Click on Term to Read More classification scale, one that is based on the total abundance of phyllosilicates in a meteorite and that does not imply an evolutionary progression from lower to higher degrees of aqueous alteration. In their scheme, phyllosilicate abundance increases along the type sequence: 3.0 (0%) ⇒ 2.0 (50%) ⇒ 1.0 (100%), where the phyllosilicate abundance increases in 0.1 (5%) increments. This method allows for a consistent scale to be applied across all carbonaceous chondrite groups. A hydration indexed chart of 40 carbonaceous chondrites, comprising CI, CM, and CR group members resolved by phyllosilicate abundance, was published in an abstract by Howard and Alexander (2013), while the classifications of 54 meteorites was published in an article by Alexander et al. titled ‘The classification of CM and CR chondrites using bulk H, C and N abundances and isotopic compositions’, GCA, vol. 123 (2013), which was followed by an article by Howard et al. titled ‘Classification of hydrous meteorites (CR, CM and C2 ungrouped) by phyllosilicate fraction: PSD-XRD modal mineralogy and planetesimal environments’, GCA, vol. 149 (2015).CLASSIFICATION BASED ON HYDRATION Adapted from Alexander et al. (2013) |
|||
---|---|---|---|
ALH 83100 | 1.1 | DOM 03183 | 1.6 |
ALH 84034 | 1.1 | GRA 98074 | 1.6 |
DOM 08003 | 1.1 | GRO 95566 | 1.6 |
Nagoya II | 1.1 | LAP 02336 | 1.6 |
SCO 06043 | 1.1 | LAP 03718 | 1.6 |
ALH 84029 | 1.2 | LEW 88001 | 1.6 |
ALH 84042 | 1.2 | LEW 90500 | 1.6 |
ALH 84044 | 1.2 | Mighei | 1.6 |
MET 01070 | 1.2 | Murchison | 1.6 |
SCO 06014 | 1.2 | Nogoya | 1.6 |
SCO 06043 II | 1.2 | Banten | 1.7 |
Cold Bokkeveld | 1.3 | GRA 98005 | 1.7 |
EET 96006 | 1.3 | LAP 02239 | 1.7 |
LAP 03785 | 1.3 | MAC 88100 | 1.7 |
LEW 87148 | 1.3 | MET 00432 | 1.7 |
ALH 85013 | 1.4 | QUE 97990 | 1.7 |
EET 96016 | 1.4 | DNG 06004 | 1.8 |
LEW 87022 | 1.4 | DOM 08013 | 1.8 |
MAC 88101 | 1.4 | LEW 85312 | 1.8 |
MAC 88176 | 1.4 | LON 94102 | 1.8 |
LAP 02277 | 1.4 | MCY 05230 | 1.8 |
QUE 93005 | 1.4 | PCA 91084 | 1.8 |
LAP 02333 | 1.5 | Bells (W) | 1.9 |
LEW 87016 | 1.5 | LEW 85311 | 1.9 |
MET 01072 | 1.5 | TIL 91722 | 1.9 |
MET 01075 | 1.5 | Bells (C) | 2.3 |
In further studies of hydrous alteration in carbonaceous chondrite groups, Howard et al. (2013) ascertained that neither the relative abundances of Fe- and Mg-rich serpentines, nor the bulk O-isotopic compositions, show any correlation to the degree of aqueous alteration. The investigators suggest that the Fe:Mg ratios in serpentines, as well as the final bulk O-isotopic compositions, were controlled instead by factors related to the anhydrous precursor material, e.g., the initial chondrule:matrix ratio, where the matrix is intrinsically more Fe-rich. Another controlling factor they invoke is the temporal abundance of water ice.
Murchison contains water-bearing minerals including serpentines. These phyllosilicates provide a high water content of 4–18 wt%, water that initially condensed at a distance of 4 AUThe astronomical unit for length is described as the "mean" distance (average of aphelion and perihelion distances) between the Earth and the Sun. Though most references state the value for 1 AU to be approximately 150 million kilometers, the currently accepted precise value for the AU is 149,597,870.66 km. The Click on Term to Read More from the SunOur parent star. The structure of Sun's interior is the result of the hydrostatic equilibrium between gravity and the pressure of the gas. The interior consists of three shells: the core, radiative region, and convective region. Image source: http://eclipse99.nasa.gov/pages/SunActiv.html. The core is the hot, dense central region in which the (Eiler and Kitchen, 2004). A total of 2.8 wt% of this water is adsorbed or trapped inside pores (Yoldi-Martinez et al., 2011). In Murchison, Mg-rich serpentines as well as Fe-rich cronstedite are found to contain –OH. Fe-rich aureoles are among the products resulting from in situ aqueous alteration processes. Additionally, Murchison contains carbonElement commonly found in meteorites, it occurs in several structural forms (polymorphs). All polymorphs are shown to the left with * indicating that it been found in meteorites and impact structures: a. diamond*; b. graphite*; c. lonsdalite*; d. buckminsterfullerene* (C60); e. C540; f. C70; g. amorphous carbon; h. carbon nanotube*. Click on Term to Read More (2–2.5 wt%) and nitrogenPrincipal constituent of the Earth’s atmosphere (78.08 vol. % at ground level). Nitrogen is the fifth most abundant element in the universe by atom abundance. Nitrogen comprises only 3.5 vol. % of the atmosphere of Venus and 2.7 vol. % of Mars’s atmosphere. Nitrogen has two isotopes: 14N (99.632 %) and 15N Click on Term to Read More (0.09–0.16 wt%) as constituents of free organicPertaining to C-containing compounds. Organic compounds can be formed by both biological and non-biological (abiotic) processes. Click on Term to Read More matter, diamondOne of the naturally occurring forms of carbon found in meteorites. Each C atom is bonded through covalent sp3 hydrid orbitals to four others. The strength of the C-C bonds makes diamond the hardest naturally occurring substance (according to the Mohs scale) in terms of resistance to scratching. There are Click on Term to Read More, and soluble complex macromolecular organic compounds (10–15 ring polycyclic aromatic hydrocarbons [PAHs]), including at least 80 amino acids (~14,000 ppbParts per billion (109). Click on Term to Read More for C2–C5) and the bases that make up the biological coding elements of RNA and DNA. All of these organic compounds have a nonbiogenic origin, and were formed by such processes as irradiation of interstellar organic ices by cosmic raysHigh-energy subatomic particles mainly originating outside the Solar System that continuously bombard the Earth from all directions. They represent one of the few direct samples of matter from outside our solar system and travel through space at nearly the speed of light. These charged particles – positively charged protons or Click on Term to Read More. A listing of amino acids identified in Murchison before 1991 can be found on the Murchison organics page. Further studies at the University of Bremen in Germany have led to the identification of seven diamino acids, which are the building blocks of peptide nucleic acids considered to have preceded RNA and DNA in the genesis of life. Also having an abioticNon-biological in origin, or not derived from living organisms. Click on Term to Read More origin, a suite of over fifty monocarboxylic acids have been identified in Murchison (Huang et al., 2004), some of which have also been found in the C2 ungrouped chondrites Tagish Lake (Herd, University of Alberta, 2009) and EET 96029, the latter containing a high abundance of formic acid. Studies of Murchison at the University of California at Davis (D. Deamer) have revealed the existence of lipid-like organic chemicals able to self-assemble into a membrane-like film enclosing a fluid, an analog to a cell membrane. Although CM chondrites with lower petrologic types (extensive aqueous alteration) contain significantly less abundant amino acids compared to those with higher petrologic types, the finding of similar relative abundances among them suggests that a common parent body link may exist (Botta et al, 2007). The case has been made, based on O-isotopic compositions, for the pre-terrestrial production of water-soluble sulfate by the oxidation of sulfides in the presence of water (Airieau, et al., 2005). This process occurred as fluid flowed through unaltered rock that still preserved a component of its original oxygenElement that makes up 20.95 vol. % of the Earth's atmosphere at ground level, 89 wt. % of seawater and 46.6 wt. % (94 vol. %) of Earth's crust. It appears to be the third most abundant element in the universe (after H and He), but has an abundance only Click on Term to Read More ratio. This sulfate is isotopically stable, and preserves the oxygen isotopic signature of the water that was present at the time of sulfate formation, and to some degree may also reflect the signature of the water that was present during aqueous alteration processes of the meteorite. The isotopic composition of Murchison and other CM chondrites reflects contributions from two primary reservoirs: 1) an anhydrous silicate component similar to the primitive CO3.0 chondrite ALHA77307, and 2) an aqueous component manifest as phyllosilicates formed through parent body processes (Clayton and Mayeda, 1999). A parent body origin for the phyllosilicates is revealed by the heavy carbon (13C) content of the carbonates, which is heavier than nebular C gas. Rather than a mass fractionationFractionation of isotopes or elements that is dependent on their masses. Click on Term to Read More process, the heavy C could have been derived from presolar carbide grains, or as proposed by Guo and Eiler (2007), through the production and escape of 13C-depleted methane during aqueous alteration. A low water/rock ratio employing isotopically-heavy water at temperatures near 0°C is considered the most likely parent body alteration environment. See the Colony page for information regarding a possible common parental source object for the CM and CO groups. Of possible historic significance is the recent discovery of a variety of sugar compounds, collectively known as polyols, within Murchison and the similar CM2 Murray. These compounds are constituents of RNA and DNA, and serve a role in cellular chemistry. Equally remarkable, fatty acids have been isolated from Murchison that independently form boundary membranes in alkaline conditions creating a rudimentary cell structure which could theoretically lead to self-replication. Two of the known nucleobases, which when associated with a sugar and a phosphate group constitute the nucleic acids that compose the genetic code, have been identified as indigenous components in Murchison (Martinsa et al, 2009): the one-ring pyrimidine, uracilOne of the five nitrogen-containing bases occurring in nucleotides., is present as a natural component of RNA, while the two-ring purine, xanthine, is instrumental in the synthesis of other purine nucleotides. Besides these discoveries, isotopic studies suggest that organic sulfur compounds within Murchison may have been created by interaction of carbon-disulfide molecules with light in the low-temperature, pre-planetary environment of interstellar space. Similarly, it was found that UV photolysis of interstellar ice could lead to the formation of the amino acids glycine, alanine, and serine, and could explain the existence of an L-enantiomer bias. In light of the fact that these naturally synthesized ingredients necessary for life are present in asteroidal material, the question arises: what influence did meteorite accumulation on Earth have on the genesis of terrestrial life? Interestingly, an ultrahigh-resolution analysis of the extraterrestrial organic matter in Murchison has revealed that its indigenous chemical diversity encompasses tens of thousands to millions of different molecular compositions, exhibiting a chemical complexity that is high compared to biological systems on Earth (Schmitt-Kopplin, 2010). Murchison also contains insoluble organic compounds. One component (20%) consists of presolar diamonds in concentrations of 1000 ppmParts per million (106). Click on Term to Read More, while another (10%) consists of grains of presolar graphiteOpaque form of carbon (C) found in some iron and ordinary chondrites and in ureilite meteorites. Each C atom is bonded to three others in a plane composed of fused hexagonal rings, just like those in aromatic hydrocarbons. The two known forms of graphite, α (hexagonal) and β (rhombohedral), have Click on Term to Read More and SiC. Croat et al. (2008) discovered that the graphite in Murchison is present as both ordered (onion types) and disordered (platy types and scaly types) morphologies. Some platy graphite grains contain internal refractory grains of oxides (e.g., eskolaite and magnetite), carbides, and RuFe-metal, similar to constituents found in onion type graphites. These platy grains also show enrichments in 12C. The combined isotopic and compositional evidence indicates that both the onion and platy graphite grains formed during s-process nucleosynthesis (in which neutrons are slowly added to nuclei over thousands of years) in one or more AGB carbon stars. It was further determined by the research team that the most disordered grain type, the scaly graphite, has O-isotopic ratios and other features more consistent with having originated during r-process nucleosynthesis occurring in supernovae. Nguyen et al. (2007) ascertained that the SiC grains in Murchison are comprised of a predominant mainstream type (from low-mass, C-rich AGB starsStars on the Asymptotic Giant Branch, which represents a late stage of stellar evolution that all stars with initial masses < 8 Msun go through. At this late stage of stellar evolution, gas and dust are lifted off the stellar surface by massive winds that transfer material to the interstellar Click on Term to Read More) plus the rare types A + B (from J-type carbon stars), X (from type II supernovae), and Y + Z (from low-metallicity AGB stars). The CRE ages of some large SiC grains range up to 1.5 b.y. The largest insoluble organic component (~70%) is a kerogen-like material (Mao, 2005). These components were mostly produced during s-process nucleosynthesis in aging, medium-sized carbon stars (TP-AGB phase), or very rarely in supernovae. TiC crystals thought to have been produced in supernovae, and rutile grains of variable composition thought to have been produced in AGB starStars on the Asymptotic Giant Branch, which represents a late stage of stellar evolution that all stars with initial masses < 8 Msun go through. At this late stage of stellar evolution, gas and dust are lifted off the stellar surface by massive winds that transfer material to the interstellar Click on Term to Read More outflows (Croat, 2007), along with rare SiC grains of uncertain origin (Hynes and Croat, 2007) have become internal constituents of later formed graphite grains. While one SiC grain has been determined to be from a novaStar that, over a period of a few days, becomes 103 to 104 times brighter than it was previously. Novae are observed about 10-15 times per year in the Milky Way. Click on Term to Read More, the origin of another anomalous grain highly enriched in 30Si has yet to be established. Additionally, it was discovered that the isotopic composition of some X-Type SiC grains is enhanced in heavy elements, which has given rise to the new formation theory of neutronCharge-neutral hadron with a mass of 1.6748 x 10-27 kg, equivalent to 939.573 MeV, and an intrinsic angular momentum, or spin, of ½ (in units of h/2π). The neutron is a nucleon, one of the two basic constituents of all atomic nuclei (apart from 1H, which consists of a single Click on Term to Read More burst nucleosynthesis. All of these interstellar grains, including aluminum oxide, spinelMg-Al oxide, MgAl2O4, found in CAIs., and silicon nitride are remnants of the dust cloud from which our Solar SystemThe Sun and set of objects orbiting around it including planets and their moons and rings, asteroids, comets, and meteoroids. was formed. The CM chondrites may be linked by spectral properties to the C-type, G-class asteroids 19 Fortuna and 13 Egeria, both of which are located near resonances that should be supplying fragments to Earth. On the other hand, Rubin and Bottke (2007) argue that the prevalence of CM xenolithic clasts in ordinary chondrites and HED breccias is consistent with a recent collisional fragmentation event. They believe the source to be an ~170-km C-type asteroid located in the inner asteroid beltBelt located between 2.12 and 3.3 AU from the Sun and located between the orbits of Mars and Jupiter containing the vast majority of asteroids. The asteroid belt is also termed the main asteroid belt or main belt to distinguish it from other asteroid populations in the Solar System such Click on Term to Read More which had been disrupted ~160 m.y. ago, and which now comprises the Baptistina asteroid family. The close proximity of this asteroid family to the ν6 resonance, as well as the similar orbits of the OC and HED parent bodies, support such a scenario. Nonetheless, contradictory spectroscopic data have been obtained by Reddy et al. (2009), including analysis of absorptionTransfer of energy to a medium as a particle or electromagnetic radiation passes through it. Absorption of electromagnetic radiation is the combined result of Compton scattering, σ, and photoelectric absorption, τ. It may be quantified: where, t = thickness, ρ = density, and μ = mass absorption coefficient, which combines Compton and photoelectric effects (μ = σ + τ). Click on Term to Read More features and albedoRatio of the amount of light reflected by an object and the amount of incident light. Albedo is used as a measure of the reflectivity or intrinsic brightness of an object. A white, perfectly reflecting surface has an albedo of 1.0 while a black perfectly absorbing surface would have an Click on Term to Read More values, suggesting that 298 Baptistina is more consistent with an S-type asteroid, perhaps similar to the LL chondrites, and is inconsistent with a carbonaceous chondrite. Interestingly, the recorded fall of the CM chondrite Maribo may provide a link to CometConglomeration of frozen water and gases (methane, ammonia, CO2) and silicates that that formed in the outer solar system and orbits the Sun. In recent years, the description of comets has shifted from dirty snowballs to snowy dirtballs with more dust than ice. However, the ratio is less than 10-to-1. Click on Term to Read More Encke within the Taurid complex. The very high eccentricityRatio of the distance between the foci and the major axis of an ellipse (planetary orbit). Eccentricity is: where ra = apoapsis distance and rp = periapsis distance. Click on Term to Read More derived for the pre-atmospheric orbitThe elliptical path of one body around another, typically the path of a small body around a much larger body. However, depending on the mass distribution of the objects, they may rotate around an empty spot in space • The Moon orbits around the Earth. • The Earth orbits around Click on Term to Read More of Maribo, as well as the location of the semimajor axis within the main asteroid beltBelt located between 2.12 and 3.3 AU from the Sun and located between the orbits of Mars and Jupiter containing the vast majority of asteroids. The asteroid belt is also termed the main asteroid belt or main belt to distinguish it from other asteroid populations in the Solar System such Click on Term to Read More, are nearly identical to those of Comet Encke (Haack et al., 2011). In addition, significant similarities exist in the D/H ratio between the water present in certain comets, such as Halley and Hyakutake, and that observed in carbonates and phyllosilicates present in CM condrites. Similarities also exist in the mineralogy of CM chondrites and the samples returned from Comet Wild 2, while high annealing temperatures are required in both to produce their crystalline silicate component. However, a large disparity in CRE ages exists between CM chondrites and that conjectured for the Taurid complex comets—the Murchison meteoroidSmall rocky or metallic object in orbit around the Sun (or another star). experienced a simple one-stage exposure history in transitWhen a small celestial body moves in front of a much larger one (as when Mercury or Venus appears in silhouette against the solar disk or when a satellite passes in front of Jupiter or Saturn). The shadow of a satellite may also transit the disk of its primary. to Earth, calculated to be 1.8 (±0.3) m.y., while the the Taurid complex comets are most consistent with a much more recent disruption event. Further investigations are necessary to better resolve this issue. On the other hand, a CRE age study of CM chondrites conducted by Meier et al. (2016) shows a possible relationship exists to the asteroid breakup event ~8.3 m.y. ago that formed the Ch/C/Cg-type members of the Veritas family. In addition to the large abundance of 3He-enriched interplanetary dust discovered in 8.2 m.y.-old deep-sea drill cores, ~1/6 of all CM meteorites have 21Ne-based CRE ages that are consistent with derivation from this catastrophic breakup, while others with significantly younger CRE ages could represent secondary collisions among the Veritas fragments. A significantly larger CM chondrite sampling (110) was utilized by Zolensky et al. (2017) in a CRE age and petrographic study. They contend that these samples fall into four separate CRE age groupings which may represent separate collisional events (see diagram below). They found that the CM chondrites with the youngest CRE ages have experienced the highest degrees of aqueous alteration, and that those with the oldest CRE ages are composed of a single lithology (monomict). Plausible explanations for these findings were presented considering both one- and two-parent body scenarios.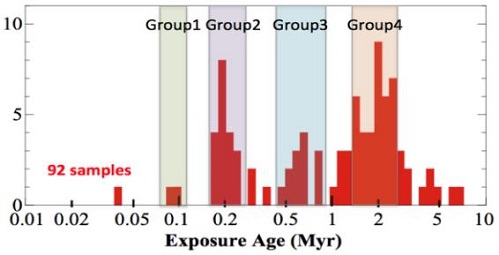
Diagram credit: Zolensky et al., 48th LPSC, #2094 (2017) and references therein Notably, a light-colored clastA mineral or rock fragment embedded in another rock. Click on Term to Read More with affinities to R chondrites was identified in a Murchison sample (Isa et al., 2013, 2014). Further analyses of this clast were conducted by Bischoff et al. (2018). The clast has a recrystallized texture with 120° triple junctions, and it was determined that both the geochemical and O-isotopic composition was similar to CM chondrites rather than to the R chondrite or brachinite groups (see diagram below). A precise determination of the parental origin for this clast is still underway.
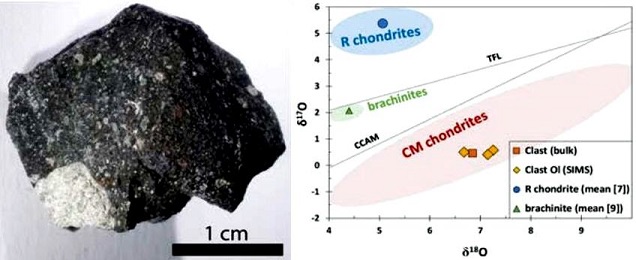
Image and diagram credit: Bischoff et al., 81st MetSoc, #6217 (2018) The specimen of Murchison shown above is a 3.1 g cut fragment exhibiting a portion of fresh black fusion crustMelted exterior of a meteorite that forms when it passes through Earth’s atmosphere. Friction with the air will raise a meteorite’s surface temperature upwards of 4800 K (8180 °F) and will melt (ablate) the surface minerals and flow backwards over the surface as shown in the Lafayette meteorite photograph below. Click on Term to Read More.