EucriteMost common type of achondrite meteorite and a member of the HED group. Eucrites are basalts composed primarily of pigeonite and anorthite (An60-98). Eucrites have been placed into three subgroups based on mineralogical and chemical differences. • Non-cumulate eucrites represent the upper crust that solidified on a magma ocean after Click on Term to Read More
Polymict, crystalline

Fell October 1960
26° 27′ S., 120° 22′ E.
This
fallMeteorite seen to fall. Such meteorites are usually collected soon after falling and are not affected by terrestrial weathering (Weathering = 0). Beginning in 2014 (date needs confirmation), the NomComm adopted the use of the terms "probable fall" and "confirmed fall" to provide better insight into the meteorite's history. If Click on Term to Read More, occurring around 1:00 P.M., was witnessed by two station workers who were opening a fence gate on the Millbillillie–Jundee track in Western Australia; however, no specimens were recovered until 1970. Searches by local Aboriginies have produced many well-preserved, oriented specimens with the shiny black
fusion crustMelted exterior of a meteorite that forms when it passes through Earth’s atmosphere. Friction with the air will raise a meteorite’s surface temperature upwards of 4800 K (8180 °F) and will melt (ablate) the surface minerals and flow backwards over the surface as shown in the Lafayette meteorite photograph below. Click on Term to Read More that is characteristic of these calcium-rich meteorites. As with most
eucritesMost common type of achondrite meteorite and a member of the HED group. Eucrites are basalts composed primarily of pigeonite and anorthite (An60-98). Eucrites have been placed into three subgroups based on mineralogical and chemical differences. • Non-cumulate eucrites represent the upper crust that solidified on a magma ocean after Click on Term to Read More, these stones contain calcium-bearing minerals such as
plagioclaseAlso referred to as the plagioclase feldspar series. Plagioclase is a common rock-forming series of feldspar minerals containing a continuous solid solution of calcium and sodium: (Na1-x,Cax)(Alx+1,Si1-x)Si2O8 where x = 0 to 1. The Ca-rich end-member is called anorthite (pure anorthite has formula: CaAl2Si2O8) and the Na-rich end-member is albite Click on Term to Read More and
augiteHigh-Ca clinopyroxene, (Ca,Mg,Fe)SiO3, that occurs in many igneous rocks, particularly those of basaltic composition. In order to be considered augite, the clinopyroxene must contain 20 to 45 mol % of calcium (Wo20 - 45). An important and unique Martian meteorite is NWA 8159, that has been classified as an augite basalt. Click on Term to Read More, which, when mixed with free
metalElement that readily forms cations and has metallic bonds; sometimes said to be similar to a cation in a cloud of electrons. The metals are one of the three groups of elements as distinguished by their ionization and bonding properties, along with the metalloids and nonmetals. A diagonal line drawn Click on Term to Read More that has been
oxidizedOxidation and reduction together are called redox (reduction and oxidation) and generally characterized by the transfer of electrons between chemical species, like molecules, atoms or ions, where one species undergoes oxidation, a loss of electrons, while another species undergoes reduction, a gain of electrons. This transfer of electrons between reactants Click on Term to Read More to
magnetiteFe oxide, Fe2+Fe3+2O4, containing oxidized iron (Fe3+) found in the matrix of carbonaceous chondrites and as diagnostic component in CK chondrites. In CK chondrites, magnetite is typically chromian, containing several wt. % Cr2O3. Click on Term to Read More, and then rapidly quenched from the molten state, produces a black, glossy
fusionProcess in which two lighter atomic nuclei combine to form a heavier atomic nucleus. Very high temperatures are normally required in order for atomic nuclei to collide with sufficient energy to overcome the Coulomb barrier (their mutual electrostatic repulsions). Fusion that occurs under high-temperature conditions is called thermonuclear fusion. Fusion Click on Term to Read More crustOutermost layer of a differentiated planet, asteroid or moon, usually consisting of silicate rock and extending no more than 10s of km from the surface. The term is also applied to icy bodies, in which case it is composed of ices, frozen gases, and accumulated meteoritic material. On Earth, the Click on Term to Read More (O.R. Norton, 2002). Millbillillie is among the most thermally equilibrated of the eucrites, a type 6 in the
metamorphicRocks that have recrystallized in a solid state due to changes in temperature, pressure, and chemical environment. Click on Term to Read More sequence of Takeda and Graham (1991). It contains a mixture of granulitic fragmental breccias and impact-melts, with a network of glassy veins and other shock features. It has been classified as a recrystallized
polymict brecciaGeneral term for all breccias that are neither monomict nor dimict. Modified from image source: http://www.saharamet.com/meteorite/gallery/HED/index.html. containing lithologies of differing Mg# (Mg/Mg+Fe) (Yamaguchi
et al., 1994).
In their paper,
Not All Eucrites Are Monomict Breccias, Yamaguchi
et al. (1997) concluded that Millbillillie, Sioux County, and several other eucrites previously believed to be monomict breccias are instead metamorphosed polymict eucrites. The variability in the
clastA mineral or rock fragment embedded in another rock. Click on Term to Read More textures suggests that many eucrites including Millbillillie are actually metamorphosed polymict breccias with a low abundance of exotic components. Additional evidence for this fact in Millbillillie is the presence of lithologies with significantly different Mg#, a likely result of
brecciationThe formation of a breccia through a process by which rock fragments of of various types are recemented or fused together. Click on Term to Read More events, probably at the floor of an
impact craterCrater formed by high-speed impact of a meteoroid, asteroid, or comet on a solid surface. Craters are a common feature on most moons (an exception is Io), asteroids, and rocky planets, and range in size from a few cm to over 1,000 km across. There is a general morphological progression Click on Term to Read More, which was followed by homogenation and recrystallization of the clasts. Further evidence of a heterogeneous composition is the difference in
244Pu–Xe ages in the components—4.566 b.y. for the fine-grained component, and 4.507 b.y. for the coarse-grained component (Quitté
et al., 31st LPSC (2000)
#1441; Miura
et al., 1998).
Mineralogical, geochemical, and petrological evidence compiled for Millbillillie suggests a formation chronology in which melting occurred very early, ~1.2 (± 1.2) m.y. after the closure of
CAIsSub-millimeter to centimeter-sized amorphous objects found typically in carbonaceous chondrites and ranging in color from white to greyish white and even light pink. CAIs have occasionally been found in ordinary chondrites, such as the L3.00 chondrite, NWA 8276 (Sara Russell, 2016). CAIs are also known as refractory inclusions since they Click on Term to Read More (Babechuk
et al., 2010). According to Al–Mg systematics,
coreIn the context of planetary formation, the core is the central region of a large differentiated asteroid, planet or moon and made up of denser materials than the surrounding mantle and crust. For example, the cores of the Earth, the terrestrial planets and differentiated asteroids are rich in metallic iron-nickel. Click on Term to Read More segregation occurred 2.5 (± 1.2) m.y. after CAIs (Schiller
et al., 2010).
MantleMain silicate-rich zone within a planet between the crust and metallic core. The mantle accounts for 82% of Earth's volume and is composed of silicate minerals rich in Mg. The temperature of the mantle can be as high as 3,700 °C. Heat generated in the core causes convection currents in Click on Term to Read More fractionationConcentration or separation of one mineral, element, or isotope from an initially homogeneous system. Fractionation can occur as a mass-dependent or mass-independent process. Click on Term to Read More occurred ~2 m.y. later and was followed by cratering processes that caused brecciation and mixing of fractionated impact melt with lithic fragments. This was then followed by a period of thermal annealing resulting in significant equilibration of the pyroxenes. A subsequent impact produced the network of glassy veins, which was then followed by another weak impact that produced fine microcracks. It has been established by Ar–Ar dating that a significant impact event occurred ~3.55 b.y. ago, resetting the chronometer to match one of several age clusters common for eucrites.
CraterBowl-like depression ("crater" means "cup" in Latin) on the surface of a planet, moon, or asteroid. Craters range in size from a few centimeters to over 1,000 km across, and are mostly caused by impact or by volcanic activity, though some are due to cryovolcanism. Click on Term to Read More retention ages that were calculated by Schmedemann
et al. (2013) show an older cluster dated at 3.75 (+0.05/–0.21) b.y., which is linked to the formation of the Veneneia basin in the northern hemisphere. In addition, they recognized a younger cluster at 3.58 (+0.07/–0.12) b.y. which corresponds to the formation of the Rheasilvia basin, the major event thought to have spalled the Vestoids, and which also corresponds to the Ar–Ar age. These crater retention age clusters not only correspond to some of the measured HED age clusters, but also to the period of
Late Heavy BombardmentPeriod between ~4.0 to 3.8 Ga ago when the Moon and other objects in the Solar System were pounded heavily by wayward asteroids. The evidence for the Late Heavy Bombardment (LHB) includes the lunar maria basins and similar structures elsewhere, such as the Caloris Basin on Mercury and the great Click on Term to Read More that affected the Moon ~4.1–3.8 b.y. ago; however, these younger clusters attest to an extended period of bombardment on
VestaThird largest and fourth brightest asteroid; it was discovered in 1807 by Heinrich Olbers and named for the ancient Roman goddess of the hearth. 4 Vesta has a basaltic surface composition and an average density not much less than that of Mars. Evidently lava once flowed here indicating that the. Furthermore, the crater counting technique revealed a global age of ~4 b.y.
Millbillillie has a Kr–Kr-based cosmic-ray exposure (CRE) age of 23.57 (±1.87) m.y., including it within the largest of five common breakup clusters established at 5–7, 10–14, 17–25, 30–46 and 70–76 m.y.
MeteoriteWork in progress. A solid natural object reaching a planet’s surface from interplanetary space. Solid portion of a meteoroid that survives its fall to Earth, or some other body. Meteorites are classified as stony meteorites, iron meteorites, and stony-iron meteorites. These groups are further divided according to their mineralogy and Click on Term to Read More examples of each of these CRE age clusters are (in m.y.) 6.93 (±0.33) Bouvante, 12 (±2) Juvinas, 23.57 (±1.87) Millbillillie, 36.37 (±2.08) Stannern, and 65–70 HaH 286. The ~22 m.y. old impact-ejection event was evidently a particularly large one since it comprises about one-third of all HED meteorites and includes representatives of all HED meteorite types (
cumulateIgneous rock composed of crystals that have grown and accumulated (often by gravitational settling) in a cooling magma chamber. Click on Term to Read More, brecciated, unbrecciated, and polymict eucrites,
diogenitesDiogenites belong to the evolved achondrite HED group that also includes howardites and eucrites. They are named after the Greek philosopher Diogenes of Apollonia, of the 5th century BCE, who was the first to suggest that meteorites come from outer space (a realization forgotten for over 2,000 years). They are Click on Term to Read More, and howardites) ejected at that time (Wakefield
et al., 35th LPSC,
#1020 [2004]; Bogard, 2009). In addition, the ~39 m.y. old impact-ejection event was statistically significant (Cartwright
et al., 2012). Some anomalous eucrites have similar CRE age values, including 26.71 m.y. for both Pasamonte and Bunburra Rockhole, and 12.5 (±0.52) m.y. for Ibitira. In a similar CRE age study also based on Kr-systematics, Strashnov et al. (2013) have determined those ages for a group of
eucriteMost common type of achondrite meteorite and a member of the HED group. Eucrites are basalts composed primarily of pigeonite and anorthite (An60-98). Eucrites have been placed into three subgroups based on mineralogical and chemical differences. • Non-cumulate eucrites represent the upper crust that solidified on a magma ocean after Click on Term to Read More falls (including Millbillillie). Although they also concluded that the majority of these eucrites correspond to only five common impact events, which occurred within the past ~50 m.y., the clusters differed slightly from those outlined above. Their five cluster events are dated at 10.6 (±0.4), 14.4 (±0.6), 21.7 (±0.4), 25.4 (±0.4), and 37.8 (±0.6) m.y. ago.
The formation history of the howardite–eucrite–
diogeniteDiogenites belong to the evolved achondrite HED group that also includes howardites and eucrites. They are named after the Greek philosopher Diogenes of Apollonia, of the 5th century BCE, who was the first to suggest that meteorites come from outer space (a realization forgotten for over 2,000 years). They are Click on Term to Read More (HED) clan began with the early
accretionAccumulation of smaller objects into progressively larger bodies in the solar nebula leading to the eventual formation of asteroids, planetesimals and planets. The earliest accretion of the smallest particles was due to Van der Waals and electromagnetic forces. Further accretion continued by relatively low-velocity collisions of smaller bodies in the Click on Term to Read More of the parent asteroid, probably
4 Vesta, within ~1 m.y. of the first
Solar SystemThe Sun and set of objects orbiting around it including planets and their moons and rings, asteroids, comets, and meteoroids. condensates. Based on its O-isotopic signature, the precursor material for this large asteroid is calculated to have had a chondritic H/CV-like (or possibly H/CR-like) composition (Rai
et al., 2016). Within a short time the body began to melt from the heat of decay of short-lived radionuclides such as
26Al and
60Fe, in addition to impact-generated heating (John T. Wasson, 2016), eventually forming a
magma oceanCompletely molten surfaces of terrestrial planets or moons that formed soon after accretion. Samples returned by the Apollo missions provide evidence of a lunar magma ocean, crystallization of which produced a stratified Moon with a low-density crust formed by accumulation of the mineral plagioclase overlying a higher density mantle of Click on Term to Read More. Metal–
silicateThe most abundant group of minerals in Earth's crust, the structure of silicates are dominated by the silica tetrahedron, SiO44-, with metal ions occurring between tetrahedra). The mesodesmic bonds of the silicon tetrahedron allow extensive polymerization and silicates are classified according to the amount of linking that occurs between the melting,
differentiationA process by which a generally homogeneous chondritic body containing mostly metal, silicates and sulfides will melt and form distinct (differentiated) layers of different densities. When the melting process continues for a long enough period of time, the once chondritic body will re-partition into layers of different composition including Click on Term to Read More, and fractionation then occurred 4.5649 (±0.0011) b.y. ago, or ~3 m.y. after
CAISub-millimeter to centimeter-sized amorphous objects found typically in carbonaceous chondrites and ranging in color from white to greyish white and even light pink. CAIs have occasionally been found in ordinary chondrites, such as the L3.00 chondrite, NWA 8276 (Sara Russell, 2016). CAIs are also known as refractory inclusions since they Click on Term to Read More formation (based on Mn–Cr systematics; Trinquier
et al., 2008; Al–Mg systematics; Schiller
et al., 2010). Notably,
mesosideriteOne of two main types of stony-iron meteorite, the other being pallasites. Mesosiderites are a mixture of approximately 50% basaltic, gabbroic and orthopyroxenitic silicates and 50% Ni-Fe metal and sulfides. The name derives from the Greek "mesos" meaning "middle" or "half" and "sideros" for "iron;" hence "half-iron". The silicates are Click on Term to Read More clasts have similar ages within error. An alternative timeline based on the Hf–W
systemDefinable part of the universe that can be open, closed, or isolated. An open system exchanges both matter and energy with its surroundings. A closed system can only exchange energy with its surroundings; it has walls through which heat can pass. An isolated system cannot exchange energy or matter with in a model reflecting a low mantle Hf/W ratio suggests that differentiation leading to core metal segregation occurred no later than 1.2 (±1.2) m.y. after the closure of CAIs (Babechuk
et al., 2009). Late accretion occurred within 2 m.y. of CAIs, but after core formation had occurred as evidenced by the finding of highly siderophile elements still remaining in
diogeniteDiogenites belong to the evolved achondrite HED group that also includes howardites and eucrites. They are named after the Greek philosopher Diogenes of Apollonia, of the 5th century BCE, who was the first to suggest that meteorites come from outer space (a realization forgotten for over 2,000 years). They are Click on Term to Read More mantle material (Day
et al., 2012).
The metallic core of Vesta eventually attained a radius of ~55–75 km. Active convective forces in the
magmaMolten silicate (rock) beneath the surface of a planetary body or moon. When it reaches the surface, magma is called lava. Click on Term to Read More ocean promoted
equilibriumTerm used to describe physical or chemical stasis. Physical equilibrium may be divided into two types: static and dynamic. Static equilibrium occurs when the components of forces and torques acting in one direction are balanced by components of forces and torques acting in the opposite direction. A system in static Click on Term to Read More crystallizationPhysical or chemical process or action that results in the formation of regularly-shaped, -sized, and -patterned solid forms known as crystals. Click on Term to Read More conditions and initiated mantle fractionation, eventually leading to eucritic melts ~2.1 m.y. after CAIs. An olivine-rich dunite layer ~150 km thick initially crystallized around the metallic core. Recent studies of the unique 1.09 g olivine-rich meteorite QUE 93148 suggest that this might be a sample of the HED mantle layer (Goodrich and Righter, 2000; C. Floss, 2003), just as the dunitic meteorites
NWA 2968 (Bunch
et al., 2006) and MIL 03443 (D. Mittlefehldt, 2008; Greenwood
et al., 2015) are thought to be. However, due to its lower Co and Ni abundances than what would otherwise be expected for an olivine-rich mantle lithology or magma ocean cumulate, QUE 93148 could have actually originated on a distinct planetary body such as that of the main-group pallasites (Shearer
et al., 2008; Shearer
et al., 2010).
Basaltic volcanism occurred very soon after differentiation of the parent body—within a short interval commencing as early as ~7 m.y. after the formation of the Solar System, and spanning a period no longer than 17 m.y. (Misawa
et al., 2005). Micron-sized zircons, associated with
ilmeniteTi-Fe oxide, TiFeO3, found in achondrites, lunar mare basalts, and shergottites. Ilmenite forms as a primary mineral in mafic igneous rocks. It crystallizes relatively early out of a magma before most of the other minerals, and as a result, the heavier crystals of ilmenite precipitate to the bottom of the magma Click on Term to Read More, have been studied from various eucrites to obtain an accurate crystallization age. The
207Pb–
206Pb ages for these zircons of ~4.554 (±0.020) b.y. represent the crystallization ages of
extrusiveRefers to igneous rocks erupted on a planetary body's surface. Click on Term to Read More eucritic lavas. However, it has been found that this crystallization event is best dated by zircons from the eucrite Igdi since those derived from Millbillillie reflect a slightly later volcanism or disturbance at 4.543 (±0.015) b.y. (Lee
et al., 2009). Coincidentally, this eucrite thermal event occurred during the time period in which the Moon is considered to have formed—in the Giant Impact 30–110 m.y. after the beginning of the solar system (Hopkins and Mojzsis, 2012).
Next in the sequence to crystallize was a cumulate, orthopyroxene-rich, diogenite layer at least 13 km thick. Ultimately, residual liquids which were subjected to
fractional crystallizationA crystallization process in which minerals crystallizing from a magma are isolated from contact with the liquid. It is a key process in the formation of igneous rocks during the process of magmatic differentiation. Also known as crystal fractionation. Click on Term to Read More (Holzheid and Palme, 2007) were extruded onto the surface. This period of volcanism produced
basaltBasalt is the most common extrusive igneous rock on the terrestrial planets. For example, more than 90% of all volcanic rock on Earth is basalt. The term basalt is applied to most low viscosity dark silicate lavas, regardless of composition. Basalt is a mafic, extrusive and fine grained igneous rock Click on Term to Read More flows that solidified to form a thin crust ~10–15 km thick (Mayne
et al., 2008; Wasson, 2012). This basaltic crustal rock was buried in turn by continual insulating flows of
lavaHot molten or semifluid rock derived from a volcano or surface fissure from a differentiated and magmatically active parent body. Click on Term to Read More, resulting in its reheating and metamorphism and eventual formation of the Main Group–Nuevo Laredo trend eucrites. The late-stage ascent of a portion of this Main Group magma was contaminated with a crustal partial melt to become the incompatible-element-rich Stannern trend eucrites.
Some of the residual liquid, or more likely a separate REE-enriched liquid, was trapped at depths of up to ~10 km and underwent late fractionation and re-equilibration processes to produce the cumulate eucrites, dated at ~60–100 m.y. after CAI formation based on Hf–W, Sm–Nd, and Lu–Hf systematics (Touboul
et al., 2008). Thereafter, surface eucritic material was impact brecciated to form a
regolithMixture of unconsolidated rocky fragments, soil, dust and other fine granular particles blanketing the surface of a body lacking an atmosphere. Regolith is the product of "gardening" by repeated meteorite impacts, and thermal processes (such as repeated heating and cooling cycles). Click on Term to Read More, which was combined with chondritic and diogenitic clasts and lithified to form the polymict
howarditeOne type of meteorite in the HED (Howardite, Eucrite, Diogenite) achondrite group. Howardites are named after the English chemist Edward Howard (1774-1816), one of the pioneers of meteoritics. Consisting mostly of eucritic and diogenitic clasts and fragments, howardites are polymict breccias. However, they can also contain dark clasts of carbonaceous Click on Term to Read More members of the HED clan (additional classification information for the HED clan can be found on the
Kapoeta page).
Vesta has an average diameter of ~506 km, with an outer basaltic crust thin enough (~15–25 km) to have been completely excavated down to diogenitic material by an impactor ~37–44 km in diameter traveling 5.5 km/sec (Ivanov and Kamyshenkov, 2013). The resulting
impact basinVery large impact structure, typically many tens to hundreds of kilometers in diameter, consisting of one or more concentric rings of peaks. Impact basins are proportionally shallower relative to their diameter compared with simple and complex craters. Basins are subdivided into central peak, peak ring and multi-ring basins. Mare Orientale Click on Term to Read More near the south pole, named Rheasilvia (after the mother of Romulus and Remus, the mythical mother of the Vestals), is ~460 km wide and ~20 km deep. Some investigators believe this excavation event occurred ~4.48 b.y. ago, while others provide evidence for a significantly more recent event consistent with the fact that the Vestoids remain in close proximity.
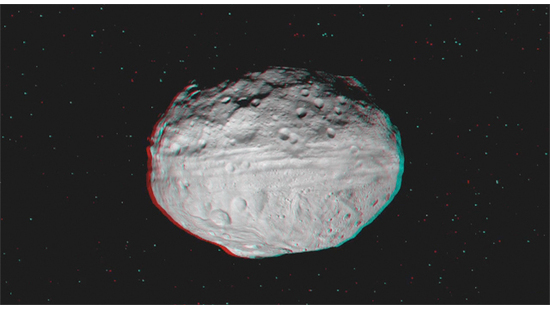
3-D image of Vesta (Anaglyph Red/Blue); credit: NASA’s Dawn Spacecraft
See the Dawn Low-Altitude Mapping Orbit-derived
Global Geologic Map of Vesta (pdf format)—Williams
et al.,
#1126 (2015) Many shallower impacts into eucrite layers also occurred between 4.1 and 3.5 b.y. ago which reset many radiometric chronometers (Bogard and Garrison, 2003). Some of the eucrite, diogenite, and howardite material was spalled into space by these impacts and was entrained deep into the 3:1 and ν
6 resonances. Searches have identified over 1,000 small (< 10 km in diameter) Vesta-like asteroids (Vestoids) composed of both eucritic and diogenitic fragments, which are thought to have been created by a late impact event 3.5 b.y. ago (Scott
et al., 2009). Some of these Vestoids bridge the gap between Vesta and the 3:1 resonance gap. From the various dynamical escape hatches, Vestoids like the near-Earth asteroids 1983 RD, 1980 PA, and 1985 DO2, were perturbed into Earth-crossing orbits on time scales of tens to hundreds of m.y.
Exposure ageTime interval that a meteoroid was an independent body in space. In other words, the time between when a meteoroid was broken off its parent body and its arrival on Earth as a meteorite - also known simply as the "exposure age." It can be estimated from the observed effects Click on Term to Read More distributions of a statistical sampling of HED meteorites show that at least two major impact events occurred around 22 m.y. and 39 m.y. ago on one or more of these Vestoids.
The basaltic eucrite Bunburra Rockhole, which was tracked by the Desert
FireballA fireball is another term for a very bright meteor, generally brighter than magnitude -4, which is about the same magnitude of the planet Venus as seen in the morning or evening sky. A bolide is a special type of fireball which explodes in a bright terminal flash at its end, often with visible fragmentation. Click on Term to Read More Network as it fell in Western Australia in 2007, was recovered during an organized field search in 2008 (Bland
et al., 2009). Its precisely calculated
orbitThe elliptical path of one body around another, typically the path of a small body around a much larger body. However, depending on the mass distribution of the objects, they may rotate around an empty spot in space • The Moon orbits around the Earth. • The Earth orbits around Click on Term to Read More is consistent with an Aten-type asteroid with a semi-major axis <1
AUThe astronomical unit for length is described as the "mean" distance (average of aphelion and perihelion distances) between the Earth and the Sun. Though most references state the value for 1 AU to be approximately 150 million kilometers, the currently accepted precise value for the AU is 149,597,870.66 km. The Click on Term to Read More. Interestingly, all petrographic characteristics studied so far are similar to the known basaltic eucrites (Spivak-Birndorf
et al., 2010). However, Bunburra Rockhole has O- and Cr-isotopic compositions distinct from that of Vesta-derived eucrites, and therefore it is thought to represent a unique basaltic parent asteroid (
e.g., Towner
et al., 2010, Benedix
et al., 2017). Bunburra Rockhole was likely delivered from the inner
main beltBelt located between 2.12 and 3.3 AU from the Sun and located between the orbits of Mars and Jupiter containing the vast majority of asteroids. The asteroid belt is also termed the main asteroid belt or main belt to distinguish it from other asteroid populations in the Solar System such Click on Term to Read More through the ν
6 secular resonance, demonstrating how material ejected from Vesta and the related Vestoids can be delivered to Earth in a like manner through an evolving orbit. Moreover, although Bunburra Rockhole and the
ungroupedModifying term used to describe meteorites that are mineralogically and/or chemically unique and defy classification into the group or sub-group they most closely resemble. Some examples include Ungrouped Achondrite (achondrite-ung), Ungrouped Chondrite (chondrite-ung), Ungrouped Iron (iron-ung), and Ungrouped Carbonaceous (C-ung). Click on Term to Read More eucrite-like
achondriteAn achondrite is a type of stony meteorite whose precursor was of chondritic origin and experienced metamorphic and igneous processes. They have a planetary or differentiated asteroidal origin where the chondritic parent body reached a sufficient size that through heating due to radioactive decay of 26Al (aluminum isotope) and gravitational Click on Term to Read More A-881394 have the same
oxygenElement that makes up 20.95 vol. % of the Earth's atmosphere at ground level, 89 wt. % of seawater and 46.6 wt. % (94 vol. %) of Earth's crust. It appears to be the third most abundant element in the universe (after H and He), but has an abundance only Click on Term to Read More and chromium
isotopeOne of two or more atoms with the same atomic number (Z), but different mass (A). For example, hydrogen has three isotopes: 1H, 2H (deuterium), and 3H (tritium). Different isotopes of a given element have different numbers of neutrons in the nucleus. Click on Term to Read More compositions, new in-depth analyses of Bunburra Rockhole conducted by Benedix
et al. (2017, and references therein) have revealed that these two meteorites have very different textures and
mineralInorganic substance that is (1) naturally occurring (but does not have a biologic or man-made origin) and formed by physical (not biological) forces with a (2) defined chemical composition of limited variation, has a (3) distinctive set of of physical properties including being a solid, and has a (4) homogeneous Click on Term to Read More chemistries;
e.g., Bunburra Rockhole has plagioclase with An
87–
90, while A-881394 has plagioclase with An
98. Based on their results, they consider it likely that these two meteorites derive from separate parent bodies. Further details about the anomalous eucrites can be found on the
Pasamonte page.
The general composition of eucrites consists of roughly equal amounts of
anorthiteRare compositional variety of plagioclase and the calcium end-member of the plagioclase feldspar mineral series with the formula CaAl2Si2O8. Anorthite is found in mafic igneous rocks such as anorthosite. Anorthite is abundant on the Moon and in lunar meteorites. However, anorthite is very rare on Earth since it weathers rapidly Click on Term to Read More, a plagioclase
feldsparAn alumino-silicate mineral containing a solid solution of calcium, sodium and potassium. Over half the Earth’s crust is composed of feldspars and due to their abundance, feldspars are used in the classification of igneous rocks. A more complete explanation can be found on the feldspar group page. Click on Term to Read More, and the clinopyroxene called
pigeoniteLow-Ca clinopyroxene, (Ca,Mg,Fe)SiO3, found as a major mineral in eucrites and shergottites. In order to be considered pigeonite, the clinopyroxene must contain 5 to 20 mol % of calcium (Wo5 - 20). Chondrites of petrologic types 4 and below contain significant low-Ca clinopyroxene. During metamorphism to higher temperatures, all existing. The source magma was probably derived from the
maficOne of the two broad categories of silicate minerals, the other being felsic, based on its magnesium (Mg) and/or iron (Fe) content. Mafic indicates silicate minerals that are predominantly comprised of Mg and/or Fe.The term is derived from those major constituents: Magnesium + Ferrum (Latin for iron) + ic (having Click on Term to Read More mineral peridotite, a mixture of
olivineGroup of silicate minerals, (Mg,Fe)2SiO4, with the compositional endpoints of forsterite (Mg2SiO4) and fayalite (Fe2SiO4). Olivine is commonly found in all chondrites within both the matrix and chondrules, achondrites including most primitive achondrites and some evolved achondrites, in pallasites as large yellow-green crystals (brown when terrestrialized), in the silicate portion Click on Term to Read More, pigeonite, and plagioclase, and is the mineral forming the bulk of the Earth’s upper mantle. The age of eucrite crystallization is ~4.5 b.y., no later than ~16.2 m.y. after the formation of Allende CAIs. Cooling and metamorphism within an
ejecta blanketGenerally symmetrical apron of ejecta surrounding a crater; it is thick at the crater's rim and thin to discontinuous at the blanket's outer edge. Click on Term to Read More lasted ~600 m.y. longer. The photo of Millbillillie above shows an 8.4 g oriented individual with radial flow lines, along with a 4.0 g partial slice showing the interior, coarse-grained texture consisting of anorthite and pigeonite.