H IMB (metallic melt brecciaWork in Progress ... A rock that is a mechanical mixture of different minerals and/or rock fragments (clasts). A breccia may also be distinguished by the origin of its clasts: (monomict breccia: monogenetic or monolithologic, and polymict breccia: polygenetic or polylithologic). The proportions of these fragments within the unbrecciated material Click on Term to Read More)
(H6 in MetBull 83)
Fell June 13, 1998
34° 10.5′ N., 103° 17.7′ W.
At 7:32 on a Saturday morning, accompanied by numerous sonic booms and smoke trails, a bright fireballA fireball is another term for a very bright meteor, generally brighter than magnitude -4, which is about the same magnitude of the planet Venus as seen in the morning or evening sky. A bolide is a special type of fireball which explodes in a bright terminal flash at its end, often with visible fragmentation. Click on Term to Read More fragmented and produced a meteorite showerThe near simultaneous fall of numerous meteorites after the fragmentation of a single asteroid or large meteoroid in the atmosphere due to the immense frictional stresses encountered during atmospheric passage. A meteorite shower will produce a strewnfield that stretch for 10s if not even 100s of km. Some famous meteorite Click on Term to Read More over eastern New Mexico. The meteoriteWork in progress. A solid natural object reaching a planet’s surface from interplanetary space. Solid portion of a meteoroid that survives its fall to Earth, or some other body. Meteorites are classified as stony meteorites, iron meteorites, and stony-iron meteorites. These groups are further divided according to their mineralogy and Click on Term to Read More was observed by a Piper Cherokee pilot and his passengers as the meteorite fell toward Portales, New Mexico. At the same time, while having their morning coffee, Nelda Wallace and Fred Stafford heard loud explosions. They ran out onto the porch in time to witness a fragment land with a thud 100 yards away, kicking up a large cloud of dust. Within a craterBowl-like depression ("crater" means "cup" in Latin) on the surface of a planet, moon, or asteroid. Craters range in size from a few centimeters to over 1,000 km across, and are mostly caused by impact or by volcanic activity, though some are due to cryovolcanism. Click on Term to Read More ten inches deep, they recovered a 16.5 kg, metal-veined fragment. In another case, a 530 g fragment penetrated the roof of Gayle Newberry’s barn and embedded itself in the north wall, verifying the meteorite’s southwest to northeast trajectory (on a course of ~62°).
Remarkably, famed meteorite hunter Skip Wilson heard the sonic booms and witnessed the corkscrew-shaped contrail produced by the meteorite as it approached. He later recovered several specimens from the area. Approximately 51 fragments were recovered over several weeks ranging in weight from 12 g to 34 kg (see photos below), having a combined weight of over 100 kg. The fragments delimit a strewnfield 12 km-long by 2.5 km-wide, and contrary to normal fallMeteorite seen to fall. Such meteorites are usually collected soon after falling and are not affected by terrestrial weathering (Weathering = 0). Beginning in 2014 (date needs confirmation), the NomComm adopted the use of the terms "probable fall" and "confirmed fall" to provide better insight into the meteorite's history. If Click on Term to Read More patterns, the largest fragments fell at the beginning of the strewnfield rather than at the end — a phenomenon similar to that of the Johnstown diogeniteDiogenites belong to the evolved achondrite HED group that also includes howardites and eucrites. They are named after the Greek philosopher Diogenes of Apollonia, of the 5th century BCE, who was the first to suggest that meteorites come from outer space (a realization forgotten for over 2,000 years). They are Click on Term to Read More fall. This unusual pattern can be explained by a multiple breakup in which the largest fragment is disrupted late downrange.Portales Valley is an unusual recrystallized, H chondriteOrdinary chondrites with a high content of free Ni-Fe metal (15-19 vol. %) and attracted easily to a magnet. Their main minerals are olivine (Fa16-20) and the orthopyroxene bronzite (Fs14.5-18.5), earning them their older name of bronzite chondrites. Chondrules average ~0.3 mm in diameter. Comparison of the reflectance spectra of Click on Term to Read More, metallic-melt breccia—a unique classification recently proposed by A. Ruzicka et al. (2005). This meteorite contains a low abundance of relict chondrulesRoughly spherical aggregate of coarse crystals formed from the rapid cooling and solidification of a melt at ~1400 ° C. Large numbers of chondrules are found in all chondrites except for the CI group of carbonaceous chondrites. Chondrules are typically 0.5-2 mm in diameter and are usually composed of olivine Click on Term to Read More (2.6 vol%) and large, mm- to cm-sized, metal-rich veins exhibiting a fine-to-medium Thomson (Widmanstätten) structure, the first chondriteChondrites are the most common meteorites accounting for ~84% of falls. Chondrites are comprised mostly of Fe- and Mg-bearing silicate minerals (found in both chondrules and fine grained matrix), reduced Fe/Ni metal (found in various states like large blebs, small grains and/or even chondrule rims), and various refractory inclusions (such Click on Term to Read More found to contain this feature (see photo below). The large metal-rich veins have a composition similar to H-chondrite metalElement that readily forms cations and has metallic bonds; sometimes said to be similar to a cation in a cloud of electrons. The metals are one of the three groups of elements as distinguished by their ionization and bonding properties, along with the metalloids and nonmetals. A diagonal line drawn Click on Term to Read More with calculated Re–Os and U–Pb ages of formation of 4.56 b.y. An absolute I–Xe age, calculated relative to Shallowater (4,562.3 [±0.4] m.y.), was calculated to be 4,559.9 (±0.5) m.y. (Bogard and Garrison, 2009). The Ar–Ar age, representing the last significant heating event, is calculated to be 4,477 (±11) m.y. The chondritic component of Portales Valley is depleted in metal (4.4%) compared to normal H-chondrite material (15–19%), suggesting that the metal-rich veins might be derived from the host silicateThe most abundant group of minerals in Earth's crust, the structure of silicates are dominated by the silica tetrahedron, SiO44-, with metal ions occurring between tetrahedra). The mesodesmic bonds of the silicon tetrahedron allow extensive polymerization and silicates are classified according to the amount of linking that occurs between the component through impact mobilization of an already hot to partially molten source.
Studies of sub-mm-sized feeder veins and portions of silicate-rich material reveal evidence for a significant heating and geochemical fractionationConcentration or separation of one mineral, element, or isotope from an initially homogeneous system. Fractionation can occur as a mass-dependent or mass-independent process. Click on Term to Read More event, probably by an endogenous heat source. This may account for the major depletion of Sm–Nd and the significant LREE enrichment. A model which best expains the trace elementSubstance composed of atoms, each of which has the same atomic number (Z) and chemical properties. The chemical properties of an element are determined by the arrangement of the electrons in the various shells (specified by their quantum number) that surround the nucleus. In a neutral atom, the number of Click on Term to Read More data favors equilibriumTerm used to describe physical or chemical stasis. Physical equilibrium may be divided into two types: static and dynamic. Static equilibrium occurs when the components of forces and torques acting in one direction are balanced by components of forces and torques acting in the opposite direction. A system in static Click on Term to Read More partial meltingAn igneous process whereby rocks melt and the resulting magma is comprised of the remaining partially melted rock (sometimes called restite) and a liquid whose composition differs from the original rock. Partial melting occurs because nearly all rocks are made up of different minerals, each of which has a different melting Click on Term to Read More with incomplete separation of melt from solid, and reflects variations in the proportion of melt and solid that existed within both silicate-rich and large vein areas. The degree of metallic partial melting was <~40%, while the silicate melt fraction was ~13%, corresponding to temperatures of ~940–1150°C. Portales Valley is the first documented metallic-melt breccia, presumed to have been created through partial melting and mobilization of the metal phase. As such, it was proposed by Ruzicka et al. that it could represent a new type of meteorite—a portalesite. Other meteorites were also formed as a result of impact processing on the H-chondrite parent bodyThe body from which a meteorite or meteoroid was derived prior to its ejection. Some parent bodies were destroyed early in the formation of our Solar System, while others like the asteroid 4-Vesta and Mars are still observable today. Click on Term to Read More, including the FeNi- metal meteorites Sacramento Wash 005 and Meteorite Hills 00428. These are the first recognized Fe- and S-rich meteorites to have been formed by impact on the H-chondrite parent body; notably, both are distinct from the IIE-iron meteorite group (Schrader et al., 2010). One formation scenario presupposes that the REEOften abbreviated as “REE”, these 16 elements include (preceded by their atomic numbers): 21 scandium (Sc), 39 Yttrium (Y) and the 14 elements that comprise the lanthanides excluding 61 Promethium, an extremely rare and radioactive element. These elements show closely related geochemical behaviors associated with their filled 4f atomic orbital. Click on Term to Read More and actinidesElements 89Ac (actinium) to 103Lr (lawrencium) found in the bottom row of the inner-transition elements of the periodic table. These elements are all radio active and the heavier in the series are unstable. For these elements the 5f orbital is the filling orbital. Click on Term to Read More mechanically segregated into phosphate and metal phases. This segregation occurred simultaneously with large, metal-rich vein formation, and probably with brecciationThe formation of a breccia through a process by which rock fragments of of various types are recemented or fused together. Click on Term to Read More of the rock during an impact event ~1.16–1.85 b.y. ago (Sm–Nd). However, this young Sm–Nd age may reflect a sampling bias, or perhaps a mixture of components having discordant ratios. Other shock indicators present in Portales Valley include silicate darkening, chromite–plagioclaseAlso referred to as the plagioclase feldspar series. Plagioclase is a common rock-forming series of feldspar minerals containing a continuous solid solution of calcium and sodium: (Na1-x,Cax)(Alx+1,Si1-x)Si2O8 where x = 0 to 1. The Ca-rich end-member is called anorthite (pure anorthite has formula: CaAl2Si2O8) and the Na-rich end-member is albite Click on Term to Read More assemblages, chromiteBrownish-black oxide of chromium and iron (Cr-Fe oxide), Cr2FeO4, found in many meteorite groups. Click on Term to Read More veinlets, metallic Cu, and irregular troiliteBrass colored non-magnetic mineral of iron sulfide, FeS, found in a variety of meteorites. Click on Term to Read More grains in FeNi-metal, which are all indicative of an earlier shock stageA petrographic assessment, using features observed in minerals grains, of the degree to which a meteorite has undergone shock metamorphism. The highest stage observed in 25% of the indicator grains is used to determine the stage. Also called "shock level". Click on Term to Read More of S6 that was followed by postshock annealing to a shock stage slightly higher than S1 (Rubin, 2004). An alternative scenario was asserted by Ruzicka et al.. It invokes only a weak shock event (S1–S2, 5–10 GPa) accompanied by shear forces to mobilize already internally heated metal into vein formation, as well as to cause deformation and brecciation, which was followed by slow cooling and annealing. In their microstructure study of nonmagmatic iron meteorites, Tomkins et al. (2013) expanded upon the latter scenario, and at the same time proposed a mechanism for a more rapid coreIn the context of planetary formation, the core is the central region of a large differentiated asteroid, planet or moon and made up of denser materials than the surrounding mantle and crust. For example, the cores of the Earth, the terrestrial planets and differentiated asteroids are rich in metallic iron-nickel. Click on Term to Read More segregation process that occurred under mostly disequilibrium conditions. They presume that multiple impacts into an already hot to partially molten body led to ponding of FeNi-metal melt and mixing of metal and silicate. As a network of increasingly-smaller fractures is created by the impacts, pressure gradients are established through which melt rapidly migrates away from high-pressure, compressed regions and into low-pressure, hydraulically dilated macro and micro fractures. During this fracturing process, lower-viscosity metal–sulfide melt develops along the margins of silicate melt flows creating a lubricating effect, thus driving a more rapid melt migration. As the concentration of sulfide within the melt steadily increases through fractionation, it is drawn into the finest fractures and between grain boundaries of the silicate component through capillary action. In Portales Valley, slow cooling at depth resulted in a chalcophile enrichment of silicates and a siderophile enrichment of metal. Eventually, as the planetesimal grew large enough to become insulating to radiogenic heat, and widespread partial silicate melting took hold, these sulfide-depleted FeNi-metal melt accumulations were rapidly gravitationally extracted into a core. One incidental fact that Tomkins et al. (2013) have elucidated from their disequilibrium scenario above is that previous Hf–W-based age studies would no longer be accurate for meteorite irons derived from parent body cores. If the large FeNi-metal accumulations that were rapidly formed through deformational impacts did not have time to allow for migration of W from silicates into the metallic melt, then the core material is not constrained in time to a very early formation, generally accepted to have occurred within 1.5 m.y. of CAIsSub-millimeter to centimeter-sized amorphous objects found typically in carbonaceous chondrites and ranging in color from white to greyish white and even light pink. CAIs have occasionally been found in ordinary chondrites, such as the L3.00 chondrite, NWA 8276 (Sara Russell, 2016). CAIs are also known as refractory inclusions since they Click on Term to Read More; i.e., the W-isotopic ratios would necessarily reflect only the initial nebular ratios identical to those of CAIs, and therefore would not be a useful chronometer for core iron meteorites. An olivineGroup of silicate minerals, (Mg,Fe)2SiO4, with the compositional endpoints of forsterite (Mg2SiO4) and fayalite (Fe2SiO4). Olivine is commonly found in all chondrites within both the matrix and chondrules, achondrites including most primitive achondrites and some evolved achondrites, in pallasites as large yellow-green crystals (brown when terrestrialized), in the silicate portion Click on Term to Read More dislocation densityMass of an object divided by its volume. Density is a characteristic property of a substance (rock vs. ice, e.g.). Some substances (like gases) are easily compressible and have different densities depending on how much pressure is exerted upon them. The Sun is composed of compressible gases and is much Click on Term to Read More analysis was conducted on Portales Valley by Hutson et al. (2007), with the results suggesting that shock effects were nonuniform at the grain-scale level, and that the overall shock intensity was on the low end of the scale at S2–S3 (5–20 GPa); the meteorite subsequently experienced annealing to produce features consistent with stage S1 (Ruzicka and Hugo, 2011). The large grain size with minimal spacing results in a porosityThe volume percentage of a rock that consists of void space. Vesicular porosity is a type of porosity resulting from the presence of vesicles, or gas bubbles, in igneous rock such as the pumice presented here. Vesicular porosity is very rare in meteorites and is often associated with slag, one Click on Term to Read More of 1.12 (±0.58) vol% (M. Strait, 2010). The N-isotopic signature of fine-grained metal extracted from a silicate portion of Portales Valley was determined. This silicate metal has a N signature that is isotopically unlike that of the metal from the large veins. Instead, the N in the silicate metal is very similar to that found in IAB iron meteorites, which coincidentally, also share a similar cooling rate. It could be inferred that the silicate portion was mixed with IAB-type metal associated with an impact-melt event, while metal in the larger veins was mobilized from within the parent body, consistent with typical IIE-type FeNi-metal. It was shown through Re–Os systematics that the metal of the postulated impact melt is more recently disturbed than the metal of the large veins. Portales Valley differs from other H chondritesChondrites are the most common meteorites accounting for ~84% of falls. Chondrites are comprised mostly of Fe- and Mg-bearing silicate minerals (found in both chondrules and fine grained matrix), reduced Fe/Ni metal (found in various states like large blebs, small grains and/or even chondrule rims), and various refractory inclusions (such Click on Term to Read More in having enrichments of FeNi-metal (probably non-representative samples), troilite, and phosphate, while showing depletions of olivine, orthopyroxeneOrthorhombic, low-Ca pyroxene common in chondrites. Its compositional range runs from all Mg-rich enstatite, MgSiO3 to Fe-rich ferrosilite, FeSiO3. These end-members form an almost complete solid solution where Mg2+ substitutes for Fe2+ up to about 90 mol. % and Ca substitutes no more than ~5 mol. % (higher Ca2+ contents occur Click on Term to Read More, and plagioclase. This composition is consistent with the addition of P-rich metal into H-chondrite precursor material, which then underwent redoxOxidation and reduction together are called redox (reduction and oxidation) and generally characterized by the transfer of electrons between chemical species, like molecules, atoms or ions, where one species undergoes oxidation, a loss of electrons, while another species undergoes reduction, a gain of electrons. This transfer of electrons between reactants Click on Term to Read More reactions with clinopyroxene (oxidative) and olivine (reductive) at temperatures of ~975°C down to ~725°C to form excess phosphate (merrillite) and orthopyroxene. Other phosphate phases could have crystallized directly from P-rich metallic melts. It was proposed by Rubin et al. (2001) that the enrichment of troilite in Portales Valley occurred as a result of the condensation of a S-rich vapor, which was produced during the impact-induced formation of a metallic melt at temperatures of at least 1477°C. In a like manner, it was found by Ruzicka et al. that the excess S present in silicate-rich areas is compensated for by the S-free coarse veins. It was argued that S-enriched metal migrated out of the metallic melt contained within the coarse veins as these veins underwent crystallizationPhysical or chemical process or action that results in the formation of regularly-shaped, -sized, and -patterned solid forms known as crystals. Click on Term to Read More; at the same time, the S-enriched melt infiltrated the silicate-rich component. Consistent with this interpretation is the presence of thin troilite veinlets (conduits) that are connected to the coarse metal veins. Two cm-size graphiteOpaque form of carbon (C) found in some iron and ordinary chondrites and in ureilite meteorites. Each C atom is bonded to three others in a plane composed of fused hexagonal rings, just like those in aromatic hydrocarbons. The two known forms of graphite, α (hexagonal) and β (rhombohedral), have Click on Term to Read More nodules, previously undiscovered in any ordinary chondriteWork in Progress Ordinary chondrites (OCs) are the largest meteorite clan, comprising approximately 87% of the global collection and 78% of all falls (Meteoritical Society database 2018)1. Meteorites & the Early Solar System: page 581 section 6.1 OC of type 5 or 6 with an apparent shock stage of S1, Click on Term to Read More, have been identified in a specimen of Portales Valley. They are entirely enclosed in coarse vein FeNi-metal, and their margins are intermingled with the metal, suggesting that they were emplaced at the same time. The graphite nodules are thought to have crystallized at high temperatures from liquid metal that was enriched in disolved carbonElement commonly found in meteorites, it occurs in several structural forms (polymorphs). All polymorphs are shown to the left with * indicating that it been found in meteorites and impact structures: a. diamond*; b. graphite*; c. lonsdalite*; d. buckminsterfullerene* (C60); e. C540; f. C70; g. amorphous carbon; h. carbon nanotube*. Click on Term to Read More, incorporated during its flow through large volumes of rock. Isotopic systematics demonstrate that the metal–silicate breccia that constitutes Portales Valley was formed in an impact event early in Solar SystemThe Sun and set of objects orbiting around it including planets and their moons and rings, asteroids, comets, and meteoroids. history, 4,559.9 (±0.5) m.y. ago. It was initially rapidly quenched, followed by very slow cooling from high temperatures as a metal–silicate mixture. Very slow cooling and annealing has erased most optical evidence of shock in olivines greater than S1, but some plagioclase indicates shock stages of S2–S3, and some silicate clasts and relict chondrules contain curvilinear trails of kamaciteMore common than taenite, both taenite and kamacite are Ni-Fe alloys found in iron meteorites. Kamacite, α-(Fe,Ni), contains 4-7.5 wt% Ni, and forms large body-centered cubic crystals that appear like broad bands or beam-like structures on the etched surface of a meteorite; its name is derived from the Greek word Click on Term to Read More and chromite blebs and veinlets which are indicative of shock stages S3–S6 (Rubin et al., 2001). In addition, the high abundance of metallic Cu grains in silicate clasts attests to significant shock pressures. The inferred cooling history constrains the location of the precursor material of Portales Valley to a depth of at least 7 km under a large crater measuring ~20 km in diameter. This material was covered by an insulating blanket of brecciated fallback material. This crater would have been about 10% of the diameter of the H-chondrite parent body. Another plausible scenario that avoids the need for such an extensive accumulation of fallback material on a small planetesimal calls for the mobilization of metal during a low-velocity impact during accretionAccumulation of smaller objects into progressively larger bodies in the solar nebula leading to the eventual formation of asteroids, planetesimals and planets. The earliest accretion of the smallest particles was due to Van der Waals and electromagnetic forces. Further accretion continued by relatively low-velocity collisions of smaller bodies in the Click on Term to Read More. Subsequent accretion would result in the burial of the material at the required depth to establish an appropriately slow cooling rate (a few °C/m.y.) and associated high metamorphism. The most efficient conversion of impact kinetic energy into heat would occur on a highly porous asteroid, which is a plausible scenario for the structure of the H-chondrite parent body (note the highly porous H chondrite Sahara 98034 on this website). Texturally, Portales Valley may be transitional between the ordinary chondrites and the silicated IIE-iron group, having many similarities to the high Fe/Si, H chondrite Rose City, and the IIE-An meteorite Netschaëvo. However, Netschaëvo is more reducedOxidation and reduction together are called redox (reduction and oxidation) and generally characterized by the transfer of electrons between chemical species, like molecules, atoms or ions, where one species undergoes oxidation, a loss of electrons, while another species undergoes reduction, a gain of electrons. This transfer of electrons between reactants Click on Term to Read More than H chondrites and has a different O-isotopic plot, indicating that although it experienced a similar petrogenesis to Portales Valley, it may have been derived from a separate parent body. Other group IIE members, as well as some IAB Complex and EL-group members, share many similar features with Portales Valley and may have experienced similar formation processes. Based on a cosmic-ray exposure ageTime interval that a meteoroid was an independent body in space. In other words, the time between when a meteoroid was broken off its parent body and its arrival on Earth as a meteorite - also known simply as the "exposure age." It can be estimated from the observed effects Click on Term to Read More for Portales Valley of ~40 m.y., nuclear track densitiesMass of an object divided by its volume. Density is a characteristic property of a substance (rock vs. ice, e.g.). Some substances (like gases) are easily compressible and have different densities depending on how much pressure is exerted upon them. The Sun is composed of compressible gases and is much Click on Term to Read More are consistent with a meteoroidSmall rocky or metallic object in orbit around the Sun (or another star). diameter of at least 60 cm. The Portales Valley specimen shown above is an 11.1 g partial slice with fusion crustMelted exterior of a meteorite that forms when it passes through Earth’s atmosphere. Friction with the air will raise a meteorite’s surface temperature upwards of 4800 K (8180 °F) and will melt (ablate) the surface minerals and flow backwards over the surface as shown in the Lafayette meteorite photograph below. Click on Term to Read More, and exhibiting large angular chondritic clasts.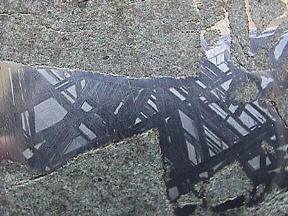
Close-up of etched metal
Photo courtesy of Michael Farmer—Mike’s Meteorites & Tektites The composite photo below shows an impressive 2,113 g etched complete slice, sectioned from the 34 kg main massLargest fragment of a meteorite, typically at the time of recovery. Meteorites are commonly cut, sliced or sometimes broken thus reducing the size of the main mass and the resulting largest specimen is called the "largest known mass". Click on Term to Read More, which is shown both in situ and after cleaning. The slice is in the collection of Dr. J. Piatek, acquired from the R. A. Langheinrich Collection, previously obtained from the finder of the mass, Robert Woolard.
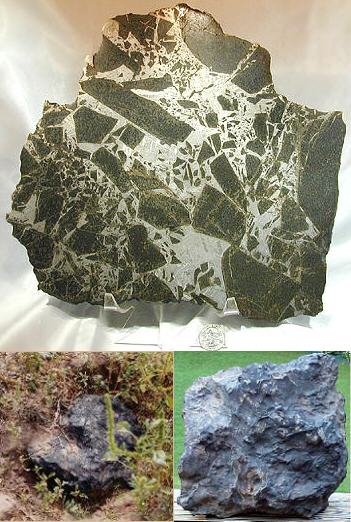