Iron, IVA, octahedriteMost Common type of iron meteorite, composed mainly of taenite and kamacite and named for the octahedral (eight-sided) shape of the kamacite crystals. When sliced, polished and etched with an acid such as nitric acid, they display a characteristic Widmanstätten pattern. Spaces between larger kamacite and taenite plates are often Click on Term to Read More
(silica-bearing)
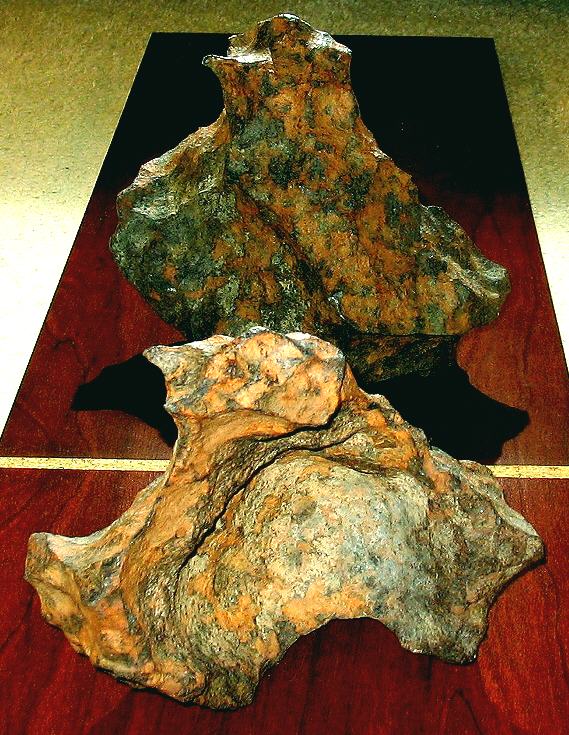
Found 1838; known before
25° 20′ S., 18° E. approx. Although the native Nama people were aware of this iron meteoriteIron meteorites consist mostly of metallic iron alloyed with typically between ~5 to ~30 wt% nickel. The main metal phases are kamacite α-(Fe, Ni) and taenite y-(Fe, Ni). Based on their group classification, they may also contain a small weight percentage of one or more of the following minerals: • Click on Term to Read More before 1836, using the metalElement that readily forms cations and has metallic bonds; sometimes said to be similar to a cation in a cloud of electrons. The metals are one of the three groups of elements as distinguished by their ionization and bonding properties, along with the metalloids and nonmetals. A diagonal line drawn Click on Term to Read More for spearheads and other weapons, it was not until this date that it was collected and described. Large masses were recovered in the Namibia Desert, Southwest Africa, in what is the largest strewnfield of any meteoriteWork in progress. A solid natural object reaching a planet’s surface from interplanetary space. Solid portion of a meteoroid that survives its fall to Earth, or some other body. Meteorites are classified as stony meteorites, iron meteorites, and stony-iron meteorites. These groups are further divided according to their mineralogy and Click on Term to Read More, covering an area of ~20,000 km². A total of over 21,400 kg have been recovered, with the largest mass of 650 kg on exhibit in the South African Museum in Cape Town. No impact craterCrater formed by high-speed impact of a meteoroid, asteroid, or comet on a solid surface. Craters are a common feature on most moons (an exception is Io), asteroids, and rocky planets, and range in size from a few cm to over 1,000 km across. There is a general morphological progression Click on Term to Read More is evident, but the severe twisting, over-folding, and cold-worked deformation of the masses attest to a violent atmospheric breakup, while the distinct regmaglypts are consistent with a long, high-velocity flight after breakup.
Gibeon is a polycrystalline octahedrite with a Ni content of 7.9% and a low P content, which exhibits a fine Thomson (Widmanstätten) structure. Along with other magmatic irons, the IVA
parent bodyThe body from which a meteorite or meteoroid was derived prior to its ejection. Some parent bodies were destroyed early in the formation of our Solar System, while others like the asteroid 4-Vesta and Mars are still observable today. Click on Term to Read More underwent
differentiationA process by which a generally homogeneous chondritic body containing mostly metal, silicates and sulfides will melt and form distinct (differentiated) layers of different densities. When the melting process continues for a long enough period of time, the once chondritic body will re-partition into layers of different composition including Click on Term to Read More, rapid cooling, and
crystallizationPhysical or chemical process or action that results in the formation of regularly-shaped, -sized, and -patterned solid forms known as crystals. Click on Term to Read More during the very early history of the
Solar SystemThe Sun and set of objects orbiting around it including planets and their moons and rings, asteroids, comets, and meteoroids., probably beginning within 1 m.y. of
CAISub-millimeter to centimeter-sized amorphous objects found typically in carbonaceous chondrites and ranging in color from white to greyish white and even light pink. CAIs have occasionally been found in ordinary chondrites, such as the L3.00 chondrite, NWA 8276 (Sara Russell, 2016). CAIs are also known as refractory inclusions since they Click on Term to Read More formation. Formation of Gibeon occurred after ~30% crystallization of the
magmaMolten silicate (rock) beneath the surface of a planetary body or moon. When it reaches the surface, magma is called lava. Click on Term to Read More, and a Pb–Pb age for a
troiliteBrass colored non-magnetic mineral of iron sulfide, FeS, found in a variety of meteorites. Click on Term to Read More inclusionFragment of foreign (xeno-) material enclosed within the primary matrix of a rock or meteorite. Click on Term to Read More in Gibeon was determined by Blichert-Toft
et al. (2011) to be 4.544 (±0.007) b.y. Similarly, the IVA
coreIn the context of planetary formation, the core is the central region of a large differentiated asteroid, planet or moon and made up of denser materials than the surrounding mantle and crust. For example, the cores of the Earth, the terrestrial planets and differentiated asteroids are rich in metallic iron-nickel. Click on Term to Read More segregation was established through the Pb–Pb chronometer from troilite in Muonionalusta to be 4.5651 (±0.0005) b.y., which is within 3 (±2) m.y. of its
accretionAccumulation of smaller objects into progressively larger bodies in the solar nebula leading to the eventual formation of asteroids, planetesimals and planets. The earliest accretion of the smallest particles was due to Van der Waals and electromagnetic forces. Further accretion continued by relatively low-velocity collisions of smaller bodies in the Click on Term to Read More (Blichert-Toft
et al., 2010) and only ~2 m.y. after CAI formation. A correction was made to the
238U/
235U value of troilite in Muonionalusta by Brennecka
et al. (2016), which led to a revision in the Pb–Pb age to 4.5584 (±0.0005) b.y.
Rare
silicaSilicon dioxide, SiO2. veins (
tridymiteSilica group mineral in which the tetrahedra occur in sheets. Tetrahedra alternately point up or down to share oxygen with tetrahedra of other sheets, forming six-sided rings perpendicular the sheets. Tridymite has a fairly open structure and accommodates Na+, K+ and Ca2+; charge balance is achieved by Al3+ ↔ Si4+.) present in both Gibeon and IVA iron Bishop Canyon were likely condensed from a cooling SiO-rich vapor that was emitted through
reductionOxidation and reduction together are called redox (reduction and oxidation) and generally characterized by the transfer of electrons between chemical species, like molecules, atoms or ions, where one species undergoes oxidation, a loss of electrons, while another species undergoes reduction, a gain of electrons. This transfer of electrons between reactants Click on Term to Read More processes. Other IVA-related iron meteorites such as Steinbach, São João Nepomuceno, and Descoberto, all of which are likely source-paired, contain an abundance of
orthopyroxeneOrthorhombic, low-Ca pyroxene common in chondrites. Its compositional range runs from all Mg-rich enstatite, MgSiO3 to Fe-rich ferrosilite, FeSiO3. These end-members form an almost complete solid solution where Mg2+ substitutes for Fe2+ up to about 90 mol. % and Ca substitutes no more than ~5 mol. % (higher Ca2+ contents occur Click on Term to Read More, tridymite, and troilite, and are considered to be products of igneous cumulates that were subsequently mixed with crystallizing metal during multiple impact events (Wasson
et al., 2006; Ruzicka, 2014).
Earlier studies of the metallographic cooling history of the IVA parent body suggested that it had a diameter of 14–98 km (Haack
et al., 1990), while another study placed the upper limit at 80 km (Wasson
et al., 2006). Moskovitz and Walker (2011) argue that the cooling rates and the U–Pb closure age of the IVA parent body are consistent with a diameter of 50–110 km after the proposed catastrophic collision. The IVA asteroid is considered to have experienced a catastrophic breakup followed by gravitational reassembly at a time when core crystallation was nearly complete. This history is consistent with the inability to successfully model the elemental trends of the core material by simple
fractional crystallizationA crystallization process in which minerals crystallizing from a magma are isolated from contact with the liquid. It is a key process in the formation of igneous rocks during the process of magmatic differentiation. Also known as crystal fractionation. Click on Term to Read More (N. Chabot, 2004), and it helps explain the widely disparate metallographic cooling rates that have been calculated, ranging from 6600°C/m.y. for the low-Ni, low-P subset, to 100°C/m.y. for the high-Ni, high-P subset. Alternatively, Moskovitz and Walker (2011) propose that the disparate cooling rates are the result of internal heating by the decay of
60Fe in an exposed core. Be that as it may, Wasson and Hoppe (2011) used a new method to determine cooling rates based on Co/Ni ratios in
kamaciteMore common than taenite, both taenite and kamacite are Ni-Fe alloys found in iron meteorites. Kamacite, α-(Fe,Ni), contains 4-7.5 wt% Ni, and forms large body-centered cubic crystals that appear like broad bands or beam-like structures on the etched surface of a meteorite; its name is derived from the Greek word Click on Term to Read More and
taeniteLess common than kamacite, both taenite and kamacite are Ni-Fe alloys found in iron meteorites. Taenite, γ-(Fe,Ni), has 27-65 wt% Ni, and forms small crystals that appear as highly reflecting thin ribbons on the etched surface of a meteorite; the name derives from the Greek word for "ribbon." Click on Term to Read More. They found no large variations in the cooling rates between two IVA irons—Bishop Canyon and Duchesne—even though they have metallographic cooling rates that differ by a factor of 25. However, Goldstein
et al. (2012) argue that these new cooling rates obtained by Wasson and Hoppe (2011) reflect inadequate spatial resolution employing a flawed phase diagram and methodology.
Using a corrected growth mechanism for kamacite, Yang
et al. (2008) calculated the cooling rate for Gibeon as 1500°C/m.y. for its Ni content of 8.04 wt%, in agreement with the finding of an inverse correlation between Ni content and cooling rate. The location of the Gibeon mass was calculated to have been ~10 km below the surface (Yang
et al., 2011). A quenching phase during formation is consistent with the observed conversion of orthopyroxene into low-Ca clinopyroxene, although this conversion could be the result of impact shock forces as well.
A scenario proposed by Wasson
et al. (2005, 2006) argues that the cooling rate estimates are correlated with the metal compositional range, and that this is best explained by a multiple-stage impact history for the IVA parent body as follows: 1) a high-velocity, high-temperature impact event onto a porous L/LL-like body melted and differentiated material within a deep
craterBowl-like depression ("crater" means "cup" in Latin) on the surface of a planet, moon, or asteroid. Craters range in size from a few centimeters to over 1,000 km across, and are mostly caused by impact or by volcanic activity, though some are due to cryovolcanism. Click on Term to Read More; 2) dissociation of FeS and
volatileSubstances which have a tendency to enter the gas phase relatively easily (by evaporation, addition of heat, etc.). loss of S, along with reduction of FeO and loss of O, produced an Fe-diluted, low-N metal magma; 3) this metallic melt drained into the core; 4) another high-temperature impact event injected a low-Ca
pyroxeneA class of silicate (SiO3) minerals that form a solid solution between iron and magnesium and can contain up to 50% calcium. Pyroxenes are important rock forming minerals and critical to understanding igneous processes. For more detailed information, please read the Pyroxene Group article found in the Meteoritics & Classification category. Click on Term to Read More/silica melt into fractures in the crystallized, hot metallic core forming the Steinbach-like assemblages; 5) a later, less energetic impact event converted orthopyroxene to clinopyroxene.
Elaborate studies integrating fractional crystallization and thermal models led some investigators (Yang
et al., 2006, 2008; Goldstein
et al., 2006, 2009; Scott
et al., 2007) to revise the formational history of the IVA parent asteroid in favor of a multi-collisional history different from that of Wasson
et al. (2005, 2006). Based on the very wide range of cooling rates inferred from the Thomson structure and from the size of the high-Ni particles within cloudy taenite zones (measuring tens of nm in size), it was determined that the IVA irons cooled on an uninsulated metallic parent object measuring ~300 km in diameter. It was ascertained that the size of high-Ni particles within cloudy taenite zones were directly correlated with the bulk Ni concentration and with the widths of outer taenite rims (
i.e., the tetrataenite region that separates the kamacite from the cloudy zone), and inversely correlated with the metallographic cooling rate (Goldstein
et al., 2008).
Through measurements of the nm-scale high-Ni particles, Goldstein
et al. (2009, 2010) concluded that this small metallic body, along with other small metallic objects, were derived from the differentiated core of an ~1,000-km-diameter protoplanetary body during a glancing collision with a larger body. During the collision, considered to have occurred 1–5 m.y. after CAI formation, the IVA body was stripped of all or most of its
silicateThe most abundant group of minerals in Earth's crust, the structure of silicates are dominated by the silica tetrahedron, SiO44-, with metal ions occurring between tetrahedra). The mesodesmic bonds of the silicon tetrahedron allow extensive polymerization and silicates are classified according to the amount of linking that occurs between the mantleMain silicate-rich zone within a planet between the crust and metallic core. The mantle accounts for 82% of Earth's volume and is composed of silicate minerals rich in Mg. The temperature of the mantle can be as high as 3,700 °C. Heat generated in the core causes convection currents in Click on Term to Read More, while extricating volatile siderophile elements such as Ge and Ga. Steinbach-like assemblages would have been formed during such a collisional disruption.
Scott
et al. (2011) envision a body that had original dimensions of >600 km when accounting for all of the differentiated layers, but which subsequently lost its silicate mantle in a glancing collision 1–2 m.y. after CAI formation. This object was then a molten metallic core 200–400 km in diameter which underwent rapid cooling. A second severe impact ~20 m.y. later produced a fragment >30 km in diameter or perhaps a rubble-pile asteroid. A final impact 400 m.y. ago delivered m-sized fragments to Earth.
The ~300-km, molten metallic body likely crystallized from the surface inwards toward the core, resulting in lower cooling rates for the more highly insulated, high-Ni, high-P iron subset. The cooling rate data of the IVA irons which have been studied place their crystallization at depths on the 300-km-diameter, uninsulated, metallic core fragment at between ~5 km (lowest Ni subset) and 90 km (highest Ni subset), the latter being about 60% of the distance to the center. On the other hand, Moskovitz and Walker (2011) combined the data of cooling rates, U–Pb closure age, and the estimated depth of the IVA Muonionalusta, and determined that the best fit was that of a 110-diameter core. They estimated that Muonionalusta formed at a core location situated 70% of its radius. In paleomagnetic studies of Steinbach and São João Nepomuceno, Bryson
et al. (2017) found evidence for a strong natural remanent magnetization in
matrixFine grained primary and silicate-rich material in chondrites that surrounds chondrules, refractory inclusions (like CAIs), breccia clasts and other constituents. Click on Term to Read More metal in Steinbach. This is consistent with the hypothesis and models of an unmantled, inwardly solidifying core that generated a dynamo (probably through non-concentric solidification) with a directionally varying field of ≥100 µT.
A second collision has been invoked to occur a few tens of m.y. after significant cooling of this 300 km-diameter metallic body, resulting in fragmentation and reassembly into a smaller body some tens of km in size. The resultant object was a heterogeneous rubble-pile comprising numerous components exhibiting a range of metallographic cooling rates. An alternative scenario has been developed by Wasson
et al. (2006) which involves multiple non-disruptive collisions.
Ultimately, an impact event involving this small agglomerate body led to the delivery to Earth of the IVA iron meteorites we now have in worldwide collections. The Gibeon
meteoroidSmall rocky or metallic object in orbit around the Sun (or another star). is calculated to have had dimensions of 4 × 4 × 1.5 m and to have entered the Earth’s atmosphere at a low angle of 10–20° from the horizon. Based on the Cl–Ar dating method, the CRE ages of the less insulated, low-Ni subset of IVA irons indicate that a catastrophic impact of the body occurred 420 (±70) m.y. ago. Secondary breakups affecting the more deeply buried, high-Ni material are reflected by two younger clusters at 255 (±15) m.y. and 207 (±13) m.y. ago. The O-isotope values of Steinbach and São João Nepomuceno are identical within analytical uncertainty to those of Gibeon and other IVA irons (Wang
et al., 2004). Moreover, these O-isotope values are similar to those of L/LL
chondritesChondrites are the most common meteorites accounting for ~84% of falls. Chondrites are comprised mostly of Fe- and Mg-bearing silicate minerals (found in both chondrules and fine grained matrix), reduced Fe/Ni metal (found in various states like large blebs, small grains and/or even chondrule rims), and various refractory inclusions (such Click on Term to Read More, which suggests that an L/LL chondritic asteroid may be the precursor to the group IVA irons. More recently, Cr-isotopic analyses for Steinbach and São João Nepomuceno conducted by Sanborn
et al. (2018) has provided further evidence for a genetic link between IVA irons and an L/LL
chondriteChondrites are the most common meteorites accounting for ~84% of falls. Chondrites are comprised mostly of Fe- and Mg-bearing silicate minerals (found in both chondrules and fine grained matrix), reduced Fe/Ni metal (found in various states like large blebs, small grains and/or even chondrule rims), and various refractory inclusions (such Click on Term to Read More parent body (see diagram below). Chromium
vs. Oxygen-isotope Plot
click on image for a magnified view
Diagram credit: Sanborn
et al., 49th LPSC,
#1780 (2018) The Appendix III contains further details about the petrogenesis of the
IVA iron group. The specimen shown above is a 6.7 kg individual (and its mirror image) with natural patina having a shape resembling a catcher’s mitt. The photo below shows one of the most spectacular Gibeon individuals in existence—a 200 kg oriented, thumbprinted mass (photo courtesy of an anonymous collector).
Dr. Svend Buhl has written a comprehensive treatise on the Gibeon meteorites, ‘
Gibeon Iron Meteorites: Discovery, History and Research‘, published on the Meteorite Recon website.